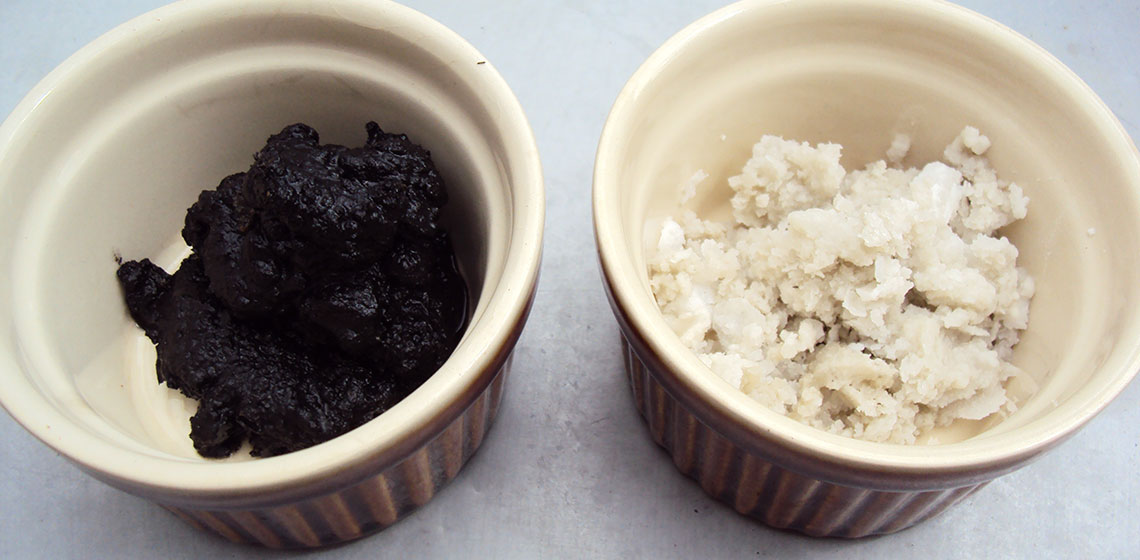
Analysis of plant remains and soils collected during excavations of the Iron Age and Roman Period salt production site at Stanford Wharf Nature Reserve on the coast of Essex have shown that saltmarsh plants and adhering sediments had been used as fuel to evaporate brine and crystallise the sea salt. It was further suggested in report of the fieldwork (Biddulph et al. 2012) that the ash of the fuel was itself a useful by-product, being steeped in the brine to create a stronger solution. The idea was not tested at the time that the report was being prepared, but in this paper, I report on experiments designed to test that hypothesis. The results show that burnt samphire (representative of saltmarsh plants), when steeped in seawater, enhances the salinity of the water, resulting in a brine that is more concentrated than one created from seawater alone. What is more, given an equal volume of brine, the brine and ash solution yields a higher amount of salt than does the seawater-only brine. The experiments support the suggestion made in the Stanford Wharf report, and have implications for the understanding of coastal salt production in the Iron Age and Roman period.
Introduction
In 2009 DP World London Gateway commissioned Oxford Archaeology to carry out an archaeological excavation of a site in the lower Thames Estuary south of the village of Stanford-Le-Hope, Essex. The work was undertaken ahead of the creation of a wetland habitat for wildlife, which was opened in 2012 as Stanford Wharf Nature Reserve, and was designed to ensure that archaeological remains were investigated before the sea wall was removed and the site flooded. The excavation uncovered extensive evidence for Middle Iron Age (circa 400-100 BC) and Roman-period (circa AD 43-410) salt manufacture and associated activities, including hearths, briquetage, ponds and channels for trapping seawater, settling tanks, and mounds of salt-production waste or 'red hills', a characteristic remnant of ancient salt production in the region (Fawn et al. 1990). The results of the excavation and subsequent analysis were published by Oxford Archaeology as monograph in 2012 (Biddulph et al. 2012).
During the post-excavation programme, soil from the red hills was treated to a battery of scientific analyses, including micromorphology, microchemistry, magnetic susceptibility, and infrared spectroscopy (Macphail et al. 2012). These revealed that the mounds, which dated to the Middle Iron Age, consisted of the ash of saltmarsh sediment and plants, which had been dried then burnt as fuel in the hearths used to evaporate brine to obtain crystallised salt. The ash was dumped, creating the mounds; the characteristic red colour derived from the burning of the marsh sediment or brickearth, which had adhered to the plants when the plants were harvested and burnt. Dumps of fuel ash in Roman-period salt-related features, including hearths and tanks, were also found to consist of burnt saltmarsh plants, although those deposits were black, rather than red, indicating that the marsh sediment had not been burnt with the plants and suggesting that the plants were more carefully harvested and processed.
Saltmarsh plants might seem an unusual and poor choice for fuel, and are not recorded to any comparable extent at other Romano-British salterns. Peat, for example, appears to have been the preferred fuel used by salters working in the Somerset Levels, where evidence of peat cutting has been found below mounds of briquetage and other salt-working waste (Hathaway 2013). In contrast, mixed assemblages of wood charcoal, cereal remains and grassland plants have been recovered from the Fenland salterns of Lincolnshire and Norfolk, although Roman-period turbaries of peat are situated close to at least one saltern (Morton Saltern) and may also have supplied some of the fuel there (Murphy 2001, 380-3). At Stanford Wharf, little wood charcoal is recorded until the late Roman period (circa. AD 250-400), its introduction coinciding with an intensification of salt production that demanded additional sources of fuel (Druce 2012). Micromorphological analysis of the marsh sediment confirms that peat was not used, although the minerogenic character of the sediment may have given the fuel a peaty quality and slowed the burning. Traces of other matter used as fuel, notably byre waste, were also detected (Macphail et al. 2012).
The principal authors of the monograph suggested that the burning of saltmarsh plants provided a useful by-product. It was proposed that the burnt plants, which would have been rich in salt, were mixed with saltwater and allowed to stand before being filtered to create a brine that was more concentrated than a brine obtained by the conventional 'open-pan' method alone. In that method, brine was obtained by trapping seawater in channels and ponds, where it was concentrated naturally in the wind and sun (Fawn et al. 1990). Both methods required the resulting brine to be evaporated over the hearth to obtain the salt. The more concentrated brine, it was argued, reduced evaporation time over the hearth, saved fuel, and increased salt yields (Biddulph et al. 2012, 81). The proposal appeared to be supported by historical and ethnographic analogies. For example, in a method known as 'sleeching', practised in Iron Age France (Daire et al. 1994, 98-9) and medieval England (McAvoy 1994), salt-impregnated sand from the shore was collected, placed into pits, then mixed with seawater. The solution was washed through a filter to create a brine. In a similar method practised in modern Tanzania, salt-impregnated sand from brackish swamps is mixed with water, and the filtered brine is evaporated (Mbogoro & Mwakipesile 2010, 10). The Western Dani of New Guinea collect porous edible plants, which are soaked in pools of salty water. The plants are extracted, then burnt over a period of several hours. The fire is doused by brine, and when the fire has cooled, small piles of crystallised salt and charcoal are collected. These are mixed with brine to create a paste, which hardens to form cakes that can be transported (Gouletquer and Weller 2015, 17-8). Peat was used to make salt in the Netherlands during the medieval period in a process called 'selnering'. Peat was cut, dried, burnt and mixed with seawater to create a brine that was evaporated (Tys 2006). Traditionally, maritime plants such as kelp, seaweed, and glassworts were dried and burnt to create soda ash or sodium carbonate and used, for example, in the manufacture of glass.
Iron Age and Roman salt-making at Stanford Wharf Nature Reserve was established within an area of upper saltmarsh on the bank of Mucking Creek in the intertidal zone of the lower Thames Estuary. Plants recorded in relative abundance in samples taken from red hills and deposits within salt-industry features and structures included sea rush (Juncus maritimus), sea plantain (Plantago maritima L.), sea lavender (Limonium sp.) and thrift (Armeria maritima (Mill.) Wild.), species characteristic of upper marsh, although species more typical of lower marsh, such as glassworts (cf. Salicornia sp.), were also present (Figure 2.27) (David 2002; Hunter 2012; Stafford and Nicholson 2012).
All saltmarsh plants are salt tolerant, and through the process of osmosis have a higher concentration of salt in their tissues compared with non-coastal plants, and a level of salinity that is equal to or higher than that of seawater (O'Donnell 2009, 41). Moreover, the upper saltmarsh typically has the highest salinity levels across saltmarsh areas, because the upper marsh is less frequently inundated by seawater than the lower marsh, and consequently during warm dry summers a greater amount of water is removed from the upper marsh sediment through evapotranspiration, increasing the concentration of salt in the soil in which the marsh plants grow (Packham and Willis 1997, 69). Both factors combined to make the site very attractive to salters wishing to use its natural resources.
Given such parallels and the properties of saltmarsh plants, the idea that such plants were used to create brine at Stanford Wharf Nature Reserve seemed a sound one in principle, but it had yet to be demonstrated in practice. In a private capacity, I designed an experiment to test the hypothesis. This paper describes the method and results of that experiment.
Method
I began my experiment in October 2014 with 100 g of fresh samphire (Salicornia europaea). The commercially available plant was easier to source than sea rush and other wild plants, and while the plant is usually found in the lower saltmarsh (including in Essex), glasswort, which is biologically identical to samphire, was identified in fuel ash deposits and had therefore been utilised by the Iron Age and Roman salters. I dried the green samphire sprigs in the open air over a period of about seven days, by which time the sprigs had turned brown and had become shrivelled with the loss of moisture. The weight of the dried plant was 30 g. To produce the ash, I burnt the dried samphire in a garden fire-bowl with a kitchen blowtorch, resulting in a pile of charred strands and powder weighing 4 g.
My next step was to create seawater. Seawater has an average salinity of 35 parts per thousand (ppt), that is, a ratio of 35 g of salt to 1000 g of water. I measured out 1 litre of household tap water, and weighed it, adjusting the amount of water fractionally to reach 1 kg. I then added 35 g of sea salt (specifically salt—that is, sodium chloride—from Le Fenouiller on the Atlantic coast of the Pays de la Loire in western France). I measured the salinity using a high precision salinity refractometer manufactured by Aqua Marin. As the salinity was a little under 35 ppt, I added more salt and measured the solution until the required value was reached.
I took three foil trays and in one (tray 1) I poured 300 ml of seawater. In tray 2, I mixed 2 g of samphire ash with 300 ml of saltwater. In tray 3, I mixed 2 g of ash with 300 ml household tap water. I placed all three trays on an internal window sill, which received light and heat through the window panes, but excluded air (See Figure 1). The order of the trays on the sill were regularly rotated in order to ensure that the trays were exposed to roughly identical ambient conditions. At approximate 24 hour intervals, I measured the salinity of water within each tray using the refractometer, which was calibrated daily using purified water and was accurate to 1 ppt. Six readings were taken for each tray were taken in total. After standing for a week, the volume of the brine from trays 1, 2 and 3 was measured (the brine in tray 2 was filtered to remove the ash) and the brine heated in a saucepan to evaporate the liquid.
Results
The readings are shown in Figure 2. The brine in tray 1 (seawater only) gained in salinity over the week, doubling from 35 ppt to 70 ppt, an increase of 100%. Conversely, the volume of liquid had halved from 300 ml to 150 ml. The salinity of the brine in tray 2 (ash and seawater) rose more dramatically from 35 ppt to 95 ppt, an increase of 171%. Meanwhile, the volume of brine reduced from 300 ml to approximately 120 ml. In tray 3, the tap water, which had a salinity reading of 0 ppt, gained salinity with the addition of ash, the level of salt increasing from 0 ppt to 11 ppt.
The brine from trays 1 and 2 was heated in succession in a saucepan to evaporate the liquid. As the liquid in trays 1 and 2 reduced in volume, a white residue (in fact an ashy grey residue in the case of the brine from tray 2) collected around the side of the pan. Crystallised salt emerged as flakes in the base of the pan when the liquid had almost evaporated completely. The salt was collected and weighed. The brine from tray 1 produced 12 g of salt, as did tray 2.
The experiment demonstrated that saltmarsh plants, in this case samphire or glasswort, enhanced the salinity of seawater when dried and burnt to ash and added to the liquid. Surprisingly, however, the ash-steeped seawater (tray 2) did not result in a higher yield of salt compared with the seawater (tray 1) alone, although the relatively small volume of brine may have accounted for this. Conducting the experiment indoors in the relatively cool month of October may also have been a factor.
To clarify these matters, the experiment was repeated, this time outdoors in July 2015 using higher quantities of ash and seawater. The method was identical to that for the first experiment, except that the conditions of the second experiment were adapted to better mimic the open conditions of the Iron Age and Roman salters. Raw samphire, this time 450 g, was dried outdoors for three days (in temperatures reaching circa 21ºC), by which time the sprigs had browned and reduced in weight to 76 g (See Figures 3 and 4). The samphire was burnt to ash, which reduced the weight further to 38 g (See Figures 5 and 6). The ash was placed in a foil tray and mixed with 1000 ml of seawater at 35 ppt. A second tray contained 1000 ml seawater only. Both trays stood outside during the day (during a heat wave, with temperatures reaching circa 35ºC) and indoors during the night (See Figure 7). After three days, the salinity of the brine in the tray containing seawater alone reached 60 ppt, an increase of 71% (See Figure 8). The salinity of the brine from the ash-steeped seawater reached 105 ppt, an increase of 200%. (This stage of the experiment could not continue to the six days of the first experiment, because the salinity of the ash-steeped brine had by three days exceeded the scale of the salinity meter.) The total volume of the ash-steeped brine was 400ml; that for the seawater alone was 550 ml, but only 400 ml of this was taken to be evaporated. The brine from both trays was then evaporated together on a gas-fired barbecue (See Figure 9). After 1.5 hours, the seawater brine had fully evaporated, leaving 44 g of crystallised salt. After 2 hours 10 minutes, the ash-steeped brine had evaporated, resulting in 70 ml of salt, although the salt differed in appearance, being black and finer (See Figure 10). After six hours, when the salt from both brines had dried completely, the respective weights were 36 g and 64 g.
Discussion
The two experiments, though conducted under slightly different conditions, demonstrated that burnt samphire (representative of saltmarsh plants), when mixed with seawater, creates a brine that after a period of natural evaporation has significantly higher salinity than that deriving from seawater alone. In addition, the second experiment showed that, given sufficient volume of seawater and amount of burnt samphire, a brine deriving from a solution of burnt plants and seawater yields a higher quantity of salt than does an equal volume of brine created from seawater alone.
Two other observations are of interest. The first, shown in both experiments, is that the rate of natural evaporation of brine in the standing trays is higher for the solution of burnt plants and seawater, compared with seawater alone, helping to increase the salinity and speeding up the period of natural brine-making. The reason is not fully understood, but it may relate to differences in the properties of the water bodies within the trays caused by the addition of burnt plants. The suspended particles are likely to affect the area of the water body surface, and depth, temperature and turbidity of the water bodies, all being factors that affect the rate of evaporation (Finch and Hall 2001, 5-8). The second observation, seen particularly in the second experiment, is that brine derived from the burnt plants and seawater solution (and with the burnt material removed), evaporates over heat at a lower rate than does the same volume of brine from seawater alone. It is a general rule, confirmed by experimental data, that the evaporation value of a liquid decreases as salinity increases (See Figure 1) (Panin and Brezgunov 2006, 664). Thus, the higher the salinity of a brine, the longer it will take to evaporate over a hearth, assuming consistent temperatures.
The second observation appears to allow us to discount the suggestion made in the Stanford Wharf volume (Biddulph et al. 2012, 81) that an advantage of using burnt saltmarsh plants to create a stronger brine was that it reduced the evaporation time required to crystallise the salt. However, given the first observation, the longer evaporation time may have been negated by the smaller volume of brine available to be evaporated in each round of production in the first instance.
Conclusion
In principal, the salters of the Iron Age and Roman salt-production site at Stanford Wharf could have used the saltmarsh plants burnt as fuel to enhance the salinity of the brine and increase the salt yield. More work is required to compare the economic cost, in terms of fuel consumption and time required, of making salt with saltmarsh plants and making salt without. It is worth noting, however, that since the ash resulting from the fuel burning was the key to the increased yields, then a longer evaporation time may itself have been a useful outcome, since it was creating a resource for the next round of salt making.
The settling tanks recorded at Stanford Wharf and associated with the Iron Age and Roman salterns (e.g. Biddulph et al. 2012: figs 6.36 & 6.40) are likely to have been used as various stages during the preparation of the brine. They held the solution of seawater and burnt saltmarsh plants and they provided a receptacle in which to pour the solution through a filter. Further filtering and a period of settling may have been necessary to clean the solution more thoroughly to avoid blackened salt, but further experimentation is required to test this. Another aspect that requires further investigation is the different evaporation rates of the various water bodies observed first during natural evaporation, and then evaporation over heat. For example, turbid water bodies (such as the brine containing the burnt plant matter) conventionally reflect more solar radiation than does clearer water, this decreasing the rate of natural evaporation (Finch and Hall 2001, 7), the opposite of what was observed in both experiments. On the other hand, the suspended charred matter may have increased the temperature of the water body, which would have increased the rate of evaporation comparative to the tray containing seawater alone. It would be interesting to run the experiment again with these and other factors more firmly in mind. The chemical properties of the salts resulting from the experiments were not established, but similarly this should be an objective of any further experimentation.
The addition of burnt saltmarsh plants to seawater to increase salt yields need not have been confined to Stanford Wharf, but may have been employed at other salt production sites in the Thames Estuary and on the Essex and Kent coasts where the utilisation of saltmarsh plants is suspected. These include Broomhey Farm in Cooling on the Hoo peninsula in north Kent, where a mound of burnt material, identified as the burnt remains of rush (Juncus maritimus), was recorded (Druce 1930), and sites recorded on the Hullbridge survey on the south coast of Essex, from which briquetage coated in a vitrified material tentatively identified as fuel ash slag derived from the combustion of monocotyledonous plant stems and leaves have been collected (Barford 1995, 175). Similar 'greenish vitreous slag' was recorded on saltern debris at Funton Marsh on the north Kent marshes (Miles 1965, 260).
The archaeological investigation at Stanford Wharf Nature Reserve demonstrated that the exploitation of saltmarsh plants was a significant, and no doubt under-recorded, aspect of Iron Age and Roman coastal salt production in Britain. The experiments described here suggest that saltmarsh plants were a fundamental resource not only as fuel, but in the production of salt itself.
Bibliography
BARFORD, P. M. 1995. The briquetage from Blackwater Site 11, in Wilkinson, T.J. and. Murphy, P.L. The archaeology of the Essex coast, volume 1: The Hullbridge survey. Chelmsford: East Anglian Archaeology 71: 173–80.
BIDDULPH, E., FOREMAN, S., STAFFORD, E., STANSBIE, D. and NICHOLSON, R. 2012. London Gateway: Iron Age and Roman salt making in the Thames Estuary. Excavation at Stanford Wharf Nature Reserve, Essex. Oxford: Oxford Archaeology Monograph 18.
DAIRE, M.-Y., GOULETQUER, P., BIZIEN-JAGLIN, C. and LANGOUET, L. 1994. La production gauloise de sel en Amorique, in Daire, M.-Y.(ed.) Le sel gauloise: Bouilleurs de sel et ateliers de briquetages amoricains à l'age du fer. Saint Malo: Les Dossiers du Centre Régional d'Archéologie d'Alet Supplément Q: 59–103.
DAVID, J. 2002. Les plantes du bord de mer. Plouédern: Editions Jean-Paul Gisserot.
DRUCE, D. 2012. Wood charcoal, in BIDDULPH, E., FOREMAN, S., STAFFORD, E. STANSBIE, D. and NICHOLSON, R. 2012. Specialist Report 20, https://library.thehumanjourney.net/909/108/20.Wood%20charcoal.pdf. Accessed 25th November 2015.
DRUCE, G.C. 1930. Report for the year ending 31st December, 1929. Archaeologia Cantiana 42: xliv–li.
FAWN, A.J., EVANS, K.A., MCMASTER, I. and DAVIES, G.M.R. 1990 The Red Hills of Essex: Salt-making in antiquity. Colchester: Colchester Archaeological Group.
FINCH, J.W. and HALL, R.W. 2001. Estimation of open water evaporation: a review of methods. R&D Technical Report W6-043/TR. Bristol: Environment Agency.
GOULETQUER, P. and WELLER, O. 2015. Techniques of salt making: from Chine (Yangtze River) to their world context. In Brigand, R. and Weller, O. (eds) Archaeology of salt: Approaching an invisible past. Leiden: Sidestone Press: 13–27.
HATHAWAY, S.-J.E. 2013. Making the invisible, visible: Iron Age and Roman salt-production in southern Britain. Doctorate Thesis, Bournemouth University, http://eprints.bournemouth.ac.uk/21381/. Accessed 23rd November 2015.
HUNTER, K. 2012. Plant macrofossils, in BIDDULPH, E., FOREMAN, S., STAFFORD, E., STANSBIE, D. and NICHOLSON, R. 2012. Specialist Report 19, https://library.thehumanjourney.net/909/116/19.Plant%20macrofossils.pdf. Accessed 6th August 2015.
MACPHAIL, R., CROWTHER, J., and BERNA, F. 2012. Soil micromorphology and microchemistry, chemistry and magnetic susceptibility, and FTIR, in BIDDULPH, E., FOREMAN, S., STAFFORD, E., STANSBIE, D. andNICHOLSON, R. 2012. Specialist Report 24, https://library.thehumanjourney.net/909/116/24.Soil%20micromorphology%20and%20chemistry.pdf. Accessed 6th August 2015.
MBOGORO, D.K. and MWAKIPESILE, A. 2010. Economical and ecological research of Bahi Swamp. Unpublished, University of Dodoma.
MCAVOY, F. 1994 Marine salt extraction: the excavation of salterns at Wainfleet St Mary, Lincolnshire. Medieval Archaeology 38: 134–63.
MILES, A. 1965 Funton Marsh, Romano-British salt panning site. Archaeologia Cantiana 80, 260–65.
MURPHY, P. 2002. Environmental studies: a general discussion, in Lane, T. and Morris, E. L. (eds.) A millennium of saltmaking : prehistoric and Romano-British salt production in the Fenland. Sleaford: Heritage Trust of Lincolnshire: 377–83.
O'DONNELL, D. 2009. Neighbourhood nature. Milton Keynes: The Open University.
PACKHAM, J.R. and WILLIS, A.J. 1997. Ecology of dunes, salt marsh and shingle. London: Chapman & Hall.
PANIN, G.N. and BREZGUNOV, V.S. 2007. Influence of the salinity of water on its evaporation, Izvestiya, Atmospheric and Oceanic Physics 43 (5): 663–665.
STAFFORD, E. and NICHOLSON, R. 2012. Landscape evolution from the late Glacial to the post-medieval period. In BIDDULPH, E., FOREMAN, S., STAFFORD, E., STANSBIE, D. and NICHOLSON, R. 2012 (Chapter 2):19–55.
TYS, D. 2006. Walraversijde, another kettle of fish? Dynamics and identity of a late medieval coastal settlement in a proto-capitalistic landscape, in Pieters, M., Verhaeghe F. and Gevaert, G.(eds.) Fishery, trade and piracy: fishermen and fishermen’s settlements in and around the North Sea area in the Middle Ages and later. Brussels: Archeologie in Vlaaderen Monografie 6: 19–40.