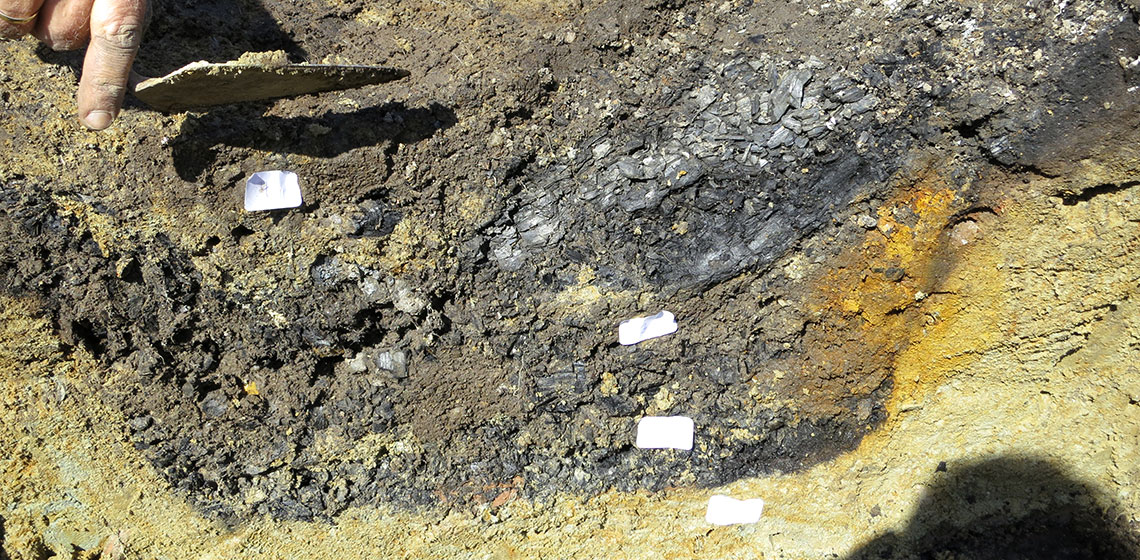
During archaeological excavations, burned bones are often found as a result of cremation, cooking or accidental fire. Even though the bones are burned, their elemental composition might still hold information about diet, habitat and health history in the past. One problem, however, is diagenesis; a range of processes changing the structure and composition of the burned bones after burial. In order to study the effects of diagenesis in burned bones, a modern domestic pig was burned and buried. Samples of the bones and the surrounding soil were taken after nine months, and again after 21 years of deposition. The samples were analyzed elemental for Al, Mn, Fe, Pb, Ca, Sr and Ba using ICP-MS, and for Hg using CV-AAS. The results indicate that Al, Mn, Fe and Ba are affected by diagenesis, whereas Pb, Hg, Sr and Ca are not.
Introduction
Bones are comprised of two tissue types, compact and trabecular. Compact bone is very dense and rigid in its structure, while trabecular bone is very spongy in appearance and brings an element of elasticity to the bone. Both types of bone tissue are composed of water, an organic part and an inorganic part (Vaughan 1975; Snyder et al. 1975). The inorganic portion is mostly composed of bioapatite a structure similar to hydroxyapatite Ca5(PO4)3(OH)2, the difference being that the phosphate (PO43-) and the hydroxyl groups (OH-) are partly substituted by carbonate groups (CO32-) (Skinner 2005). Trace elements such as Sr, Ba, Pb and Hg can substitute for Ca in the bioapatite of the bones when they are absorbed by the body via food, drinks or medicine. Chemical analysis of trace elements in bones from archaeological samples have been used by several researchers in the attempt to find information about diet, provenance, social status and health status of past human populations (Cucina et al. 2011; Ezzo 1992; Rasmussen et al. 2008; 2013a; Schutkowski and Hermann, 1999).
In this article, trace elements from bones of a burned pig are studied. When bones are heated up during the burning process, water evaporates and the organic parts combust leaving only the inorganic parts of the bones (Snoeck et al. 2014). The remaining parts of bone are usually completely white indicating that they are fully calcified and have changed in composition and structure (Stiner et al. 1995). The burning process changes the structure and concentrations of trace elements in the bones, and these alterations must be taken into consideration when drawing conclusions. Despite the changes in composition and structure, burned bones might still hold important information about past cultures.
In pig bone the elements measured can be interpreted as follows:
- Sr and Ba are present in water and feed, and are therefore dietary in origin. The concentration of Sr and Ba in the bones depends on the bioavailability of Sr and Ba in the geographical area where the feed was produced. Sr and Ba measured in the bones have the potential to provide information about the feed and habitat of the pig (Cucina et al. 2011; Ezzo 1992).
- Pollution from the environment and constituents from vaccines might contribute elements such as Pb and Hg to pig bone samples (Bak et al. 1997; EMA, 2014; Geier et al. 2007), however high concentrations of Pb and Hg in a modern-, Danish-, domestic pig is not expected because heavy metals in food stuff are closely monitored in Denmark (EC 2006). Mercury is very volatile and might diminish in the bones due to evaporation during the cremation process.
One major problem with studying archaeological bone is diagenesis. Diagenesis is postdepositional processes, which are defined as, “cumulative physical, chemical, and biological processes that alter all archaeological materials in the burial environment” (Wilson and Pollard 2002). These processes have been reviewed and will not be described in detail in this article (Hedges 2002). Research has demonstrated that the burning process itself changes the composition and structure of the bone (Snoeck and Schulting 2013), however some researchers claim that these heat induced changes make the bones better for chemical analysis than unburned bone because it makes them more resistant to diagenesis, (Snoeck et al. 2014; Zazzo and Saliège 2011).
The main objective of this project was to study the processes of diagenesis in burned, and buried pig bone. The focus was the essential element Ca and the trace elements Sr, Ba, Hg, and Pb. In addition, Al, Mn, and Fe were also measured. It is believed that high concentrations of Al, Mn and Fe in bones are a result of diagenetic processes. The presence of Al might be the result of clay minerals from the soil contaminating the bones (Rasmussen et al., 2013a). Manganese and Fe are likely to be deposited by groundwater in pores and cracks of the bones (López-González et al. 2006; Rasmussen et al. 2013a).
In order to reconstruct and study an Early Bronze Age or Late Iron Age cremation burial, a modern pig was burned and afterwards buried in 1992 (Henriksen 1993). In addition, the diagenetic processes in burned, buried bone were studied. Nine months after burial, in 1993, half of the pig was excavated, and pieces of calcified pig bone were sampled. In 2013, the other half of the pig was excavated and again fragments of pig bone were sampled. In 2013, the surrounding soil was sampled as well. The samples were analyzed for Al, Mn, Fe, Ca, Sr, Ba, Pb using ICP-MS and Hg using CV-AAS.
Methods and Sampling
In 1992 a modern-, Danish-, domestic pig was slaughtered, burned and buried, in as close of a resemblance as possible to an Early Bronze Age or Late Iron Age cremation burial. The archaeological experiment was carried out near Odense, Denmark, by the Danish archaeologist, Morgens Bo Henriksen. A detailed description of the experiment was published in 1993 (Henriksen 1993). A 30-35 kg pig was placed on a funeral pyre made of wood and barley straw. The funeral pyre, which was 1 meter in height, was placed on top of an approximately 60 cm deep pit. At the bottom, the pit was 58 cm x 52 cm. The pyre was lit, and after 21 hours, the pyre and the pig were completely combusted. The burned bone fragments and ashes had fallen into the pit, and the pit was then covered with soil and turf. In 1993, nine months after burial, half of the pig was excavated, and three pieces of the remaining bone were collected. The rest of the pig was excavated in 2013 and three additional pieces of bone were sampled (See Figure 1a and 1b).
The pieces of bone were approximately 3-4 cm in length, and due to the heat, were white, very porous, and brittle in structure. No visual differences were observed between the bones sampled in 1993 and in 2013. Bone sample AL-1, excavated in 1993, and bone sample AL-4, excavated in 2013, can be seen in Figure 1. During the excavation in 2013 the surrounding soil was sampled as well. Sample AL-10 to AL-15 were sampled from the pit and AL-16 to AL-19 were sampled at approximately 1 meter from the pit. The sampling strategy for the soil and the bones was performed as described by Rasmussen et al. (2013a; 2013f). The bone samples were taken using a Dremel MultiPro® electric drill equipped with a 2 mm drill bit. During sampling, gloves and a hairnet were worn to avoid contamination of the sample. The drill was decontaminated with distilled water and an ethanol flame between each sample. The outer layer of the bone was mechanically cleaned with the electrical drill before the sample was taken. The soil samples were taken with an in-house built handheld hollow and sharp drill of 6 mm in diameter, approximately 2 cm in length, and weighing 1–2 gram. The stainless steel tool was decontaminated with clean paper tissue between each sample. The samples were transferred to glass vials and stored in a refrigerator at approximately 5 degree ◦C. In order to homogenize each soil sample, the soil sample was placed along an in-house build aluminium-shelf and ten subsamples were randomly taken. The ten subsamples were mixed and homogenized. The utensils were cleaned with distilled water and dried with an ethanol flame after each sample.The six bone samples and the soil samples, described in Table 1, were analysed with ICP-MS and CV-AAS.
Sample name | Structure | Sample location | Sampled year |
Bone standard | Archaeological bone | ||
Bone Meal | Modern bone | ||
Apple leaf | Apple leaf | ||
AL-1 | Bone from modern pig | From area of fire | 1993 |
AL-2 | Bone from modern pig | From area of fire | 1993 |
AL-3 | Bone from modern pig | From area of fire | 1993 |
AL-4 | Bone from modern pig | From area of fire | 2013 |
AL-5 | Bone from modern pig | From area of fire | 2013 |
AL-6 | Bone from modern pig | From area of fire | 2013 |
AL-10 | Soil | From area of fire circa 50 cm in depth | 2013 |
AL-11 | Soil | From area of fire circa 35 cm in depth | 2013 |
AL-12 | Soil | From area of fire circa 20 cm in depth | 2013 |
AL-13 | Soil | From area of fire circa 10 cm in depth | 2013 |
AL-14 | Soil | From the surface of fire | 2013 |
AL-16 | Soil | Circa 2 m from fire | 2013 |
AL-17 | Soil | Circa 2 m from fire | 2013 |
AL-18 | Soil | Circa 2 m from fire | 2013 |
AL-19 | Soil | Circa 2 m from fire | 2013 |
Table 1. List of the samples AL-1 to AL-19 including name, description, sample location and sample year.
Analytical Techniques
ICP-MS
Aluminium, Mn, Fe, Ca, Sr, Ba and Pb were measured with ICP-MS (inductively coupled mass spectrometry) provided by the CHART group at Southern University of Denmark, and according to methods developed by the group (Skytte and Rasmussen 2013). A sample of approximately 20 mg was transferred to a new polystyrene container and dissolved in a mixture of 4 mL HNO3 and 2 mL H2O2 (ICP-MS grade). After shaking for approximately four hours, the sample was dissolved and to remove excess H2O2 0.67 mL HCl (ICP-MS grade) was added. The container was left shaking overnight at room temperature. Next day, each sample was halved. One half of the sample was measured with ICP-MS, the other half was measured with CV-AAS. The sample parts measured with ICP-MS were diluted to 10 mL with milli-Q water and filtered through a 45 µm PVDF Q-max filter. The analysis was performed on a Bruker ICP-MS 820 equipped with a frequency-matching RF generator and a Collision Reaction Interface (CRI), operating with helium or hydrogen. The CRI Reaction System was activated for Fe56 because of interferences with polyatomic species produced by a combination of isotopes coming from the argon. Fe56 was measured with hydrogen as skimmer gas. Al27, Ca44, Mn55, Sr88, Ba137 and a combination of Pb isotopes (Pb206, Pb207 and Pb208) were measured without skimmer gas. The basic parameters were as follows: radiofrequency power 1.40 kW; plasma gas flow 15.50 L min-1; auxiliary gas flow 1.65 L min-1; sheath gas flow 0.12 L min-1; nebulizer gas flow 1.00 L min-1. A mixture of Sc45, Y89 and Tb159 was used as an internal standard added to all analyses. The dwell time on each peak was 30 ms. For each sample, five replicates were made, and each replicate consisted of 30 mass scans. Multi-element calibration standards were prepared in 1% HNO3 at six different concentrations (0, 1, 10, 20, 100 and 200 μg L-1). For the main element Ca, three standards of 10, 20 and 25 weight percent were run. A certified modern bone meal standard (NIST SRM 1486) and an in-house archaeological human bone standard were measured alongside with the samples in order to monitor drift in the system. The Limits Of Quantification (LOQ) were calculated from measurements of the in-house bone standard. The LOQ values are listed in Table 2.
CV-AAS
Mercury was measured by CV-AAS (cold vapour atomic absorption spectroscopy) on a Flow Injection Mercury System (FIMS-400) a dedicated Hg analyzer by PerkinElmer provided by the CHART group at Southern University of Denmark (Rasmussen et al. 2013a). The sample was diluted to 19 mL, and 1 mL concentrated KMnO4 was added to the sample solution two hours prior to analysis. KMnO4 was added to maintain Hg in solution. The sample was introduced into the FIMS. In the reaction chamber of the FIMS-400, NaBH4 was added to release Hg as vapour. Three replicates were made for every sample. A certified apple leaf standard (NIST SRM 1515) and an in-house archaeological human bone standard were measured together with the samples in order to monitor drift in the system. The LOQ for Hg (Table 2) were calculated from measurements of the in-house bone standard.
Element | Al (µg/g) | Mn (µg/g) | Fe (µg/g) | Ca (%) | Sr (µg/g) | Ba (µg/g) | Pb (µg/g) | Hg (ng/g) |
LOQ | 1,56 | 2,22 | 15,92 | 1,37 | 3,06 | 3,52 | 0,94 | 8,72 |
Table 2.The LOQ values for Al, Mn, Fe, Ca, Sr, Ba, Pb and Hg calculated from measurements of the in-house bone standard using ICP-MS and CV-AAS.
Results
The results of the ICP-MS and the CV-AAS measurements are listed in Table 3 and graphically depicted in Figures 2, 3 and 4. Sample AL-1, AL-2 and AL-3 are bones excavated in 1993. Sample AL-4, AL-5 and AL-6 are bones excavated in 2013. AL-10 to AL-15 are soil samples from the pit and AL-16 to AL-19 are soil samples from the background. In Table 3 the concentrations of the elements are listed in µg/g for all the elements except for Ca and Hg, which are depicted in weight percent of bone and ng/g, respectively. The relative standard deviations (RSD) at ±1 ơ are expressed in percent of the concentrations. For results below the Limit Of Quantification (LOQ), the values are not given in the table. In Figures 2, 3 and 4 the sample names are listed on the x-axis, and on the y-axis the concentrations are depicted.
Sample name | Concentrations | |||||||
Al (µg/g) | Mn (µg/g) | Fe (µg/g) | Ca (wt%) | Sr (µg/g) | Ba (µg/g) | Pb (µg/g) | Hg (ng/g) | |
AL-1 (bone 1993) | 3.78 ± 0.37 | 67.1 ± 0.49 | 51.4 ± 13.4 | 36.2 ± 1.57 | 194.4 ± 0.67 | 12.6 ± 1.43 | <LOQ | <LOQ |
AL-2 (bone 1993) | 14.7 ± 1.10 | 103 ± 0.76 | 59.1 ± 16.1 | 33.3 ± 0.68 | 184.5 ± 0.56 | 17.8 ± 1.13 | <LOQ | <LOQ |
AL-3 (bone 1993) | 53.1 ± 0.56 | 117 ± 0.54 | 437 ± 5.64 | 34.2 ± 1.45 | 190 ± 0.45 | 16.3 ± 0.84 | <LOQ | <LOQ |
AL-4 (bone 2013) | 67.6 ± 0.39 | 1339 ± 0.27 | 2524 ± 5.77 | 35.3 ± 1.60 | 171.3 ± 0.34 | 101.7 ± 0.53 | <LOQ | <LOQ |
AL-5 (bone 2013) | 117 ± 0.58 | 691 ± 0.43 | 1067 ± 3.81 | 35.4 ± 0.87 | 167.9 ± 0.14 | 40.7 ± 1.16 | <LOQ | <LOQ |
AL-6 (bone 2013) | 152 ± 0.41 | 1638 ± 0.15 | 1075 ± 3.19 | 35.6 ± 1.50 | 185.4 ± 0.90 | 62.1 ± 0.98 | <LOQ | <LOQ |
AL-10 (Soil from pit) | 1504 ± 0.46 | 69.6 ± 0.52 | 4035 ± 2.32 | <LOQ | 4.66 ± 0.37 | 20.8 ± 0.88 | 6.05 ± 1.13 | 28.6 ± 8.76 |
AL-11 (Soil from pit) | 1177 ± 0.75 | 339 ± 0.55 | 3087 ± 2.29 | <LOQ | 21.4 ± 0.30 | 45.4 ± 1.12 | 5.25 ± 0.69 | 22.0 ± 13.7 |
AL-12 (Soil from pit) | 1127 ± 0.30 | 213 ± 0.56 | 3548 ± 0.91 | <LOQ | 8.07 ± 0.91 | 32.4 ± 0.43 | 13.3 ± 0.64 | 85.9± 2.99 |
AL-13 (Soil from pit) | 1551 ± 1.59 | 113 ± 1.08 | 4056 ± 1.52 | <LOQ | 6.17 ± 0.31 | 28.4 ± 1.00 | 14.2 ± 1.01 | 122.2 ± 4.15 |
AL-14 (Soil from pit) | 1339 ± 0.76 | 332 ± 0.21 | 5982 ± 1.37 | <LOQ | 9.14 ± 0.59 | 54.3 ± 0.50 | 15.2 ± 1.80 | 100.4 ± 2.37 |
AL-16 (Soil 2 m from pit) | 1609 ± 0.67 | 779 ± 0.38 | 5073 ± 1.84 | <LOQ | 10.4 ± 0.56 | 94.8 ± 0.62 | 16.0 ± 0.99 | 120.7 ± 2.33 |
AL-17 (Soil 2 m from pit) | 1548± 0.61 | 713 ± 0.44 | 6037 ± 3.14 | <LOQ | 9.81 ± 0.70 | 59.5 ± 0.47 | 18.4 ± 0.41 | 100.4 ± 3.98 |
AL-18 (Soil 2 m from pit) | 1328 ± 1.06 | 182 ± 1.69 | 4270 ± 1.49 | <LOQ | 7.02 ± 1.22 | 30.7 ± 1.26 | 16.5 ± 1.39 | 118.5 ± 3.35 |
AL-19 (Soil 2 m from pit) | 1404 ± 0.9 | 503 ± 0.54 | 4415 ± 2.03 | <LOQ | 7.03 ± 0.59 | 54.3 ± 0.78 | 19.1 ± 0.53 | 200.8 ± 1.62 |
Table 3. Results of the Al, Mn, Fe, Ca, Sr, Ba, Pb and Hg measurements made on modern-, domestic-, Danish pig bone using ICP-MS and CV-AAS.
Aluminium, Fe and Mn
The results of Al, Fe and Mn are shown in Figure 2.
Comparing the concentrations of Al and Fe in the bones and in the soil reveal that the concentrations in the soil are much higher than in the bones. It is also clear that the concentrations of Al and Fe in the bones sampled in 2013 are higher than the concentrations in the bones sampled in 1993. No difference in the Al or the Fe concentrations are observed between the soil from the pit and the background.
The results of the Mn concentrations in the bones and in the soil are different from the results of Al and Fe. The concentrations of Mn in the soil samples are higher than the concentrations in the bones sampled in 1993, but lower than the Mn concentrations in the bones sampled in 2013. As the concentrations of Al and Fe, the Mn concentrations measured in the bones sampled in 2013 are higher than the Mn concentrations measured in the bones sampled in 1993. Unlike the concentrations of Al and Fe, a difference is observed in the Mn concentration between the soil from the background and the soil from the pit. The Mn concentrations in the soil from the background is higher than in the soil from the pit.
Fig 2. Aluminium, Mn and Fe concentrations determined for bone and soil samples of AL-1 to AL-19. The sample names are listed on the x-axis and the concentrations are depicted on the y-axis. Aluminium is marked in red Mn is marked in green and Fe is marked in blue.
Mercury and Pb
The concentrations of Hg and Pb in the modern pig bone are below LOQ (Figure 3). The mean Hg concentration in the soil is 108 ± 4.33 ng/g, and the mean Pb concentration in the soil is 14.1 ± 0.96 µg/g.The Pb concentrations in soil samples AL-10 and AL-11 from the pit are lower than in the rest of the soil samples.
Fig 3. Hg and Pb concentrations determined for bone and soil samples of AL-1 to AL-19. The sample names are listed on the x-axis and the concentrations are depicted on the y-axis. Mercury is marked in red and Pb is marked in green.
Calcium, Sr and Ba
Figure 4 graphically illustrates the concentrations of Ca, Sr and Ba.
The Ca concentrations in the soil are below LOQ, but in the bones the Ca concentrations are between 33.3 ± 0.68 wt% and 36.2 ± 1.57 wt% with a mean of 35.0 wt%. No difference in the Ca concentrations between the samples excavated in 1993 and 2013 was found.
The Sr concentrations are lower in the soil than in the bones sampled in both 1993 and in 2013. No difference is observed between the Sr concentrations in the bones excavated in 1993 and 2013 or between the Sr concentrations in the soil from the pit and the background.
The Ba concentrations in the bone samples increase from 1993 to 2013, and theBa concentrations in the bones from 1993 are lower than the concentrations in the soil. No difference between the Ba concentration in the bones from 2013 and the soil was found. The Ba concentrations in the soil from the area of the pit and the background were not different.
Fig 4. Ca, Sr and Ba concentrations determined for bone and soil samples of AL-1 to AL-19.The sample names are listed on the x-axis and the concentrations are depicted on the y-axis.Calcium is marked in red Sr is marked in green and Ba is marked in blue.
Discussion
The results of this study suggest that when bones are buried in soil they become contaminated with Al, Mn, Fe and Ba from the soil. Strontium, however, seems to diffuse from the bones into the soil and calcium appears to be unchanged. The Hg and the Pb concentrations in the bones are below the Limit of Quantification and no transport of Hg or Pb into the bones was observed.
The Al concentration in the soil is much higher than the Al concentration in the bones. This is no surprise since the average, global Al concentration in soil is very high (62000 µg/g) (Rauch and Pacyna 2009), and the normal level of Al in piglet bone is 1.6 ± 0.9µg/g (Sedman et al. 1987). Since the Al concentration increase from 1993 to 2013, and theAl concentration measured in this study are much higher than reported by Sedman, it seems likely that most of the measured Al in this study is a result of soil contamination. Studies have shown that Al is removed to some extent by mechanical cleaning of the bone surface before analysis, but since Al is situated in the cracks and pores of the bones as well as on the surface, removing all Al is practically impossible (Skytte and Rasmussen 2013).
The Mn concentrations in the bones measured in this study are also much higher than the values reported forpig bones (1.59-11.09 µg/g) (Duan et al. 2013; TAO et al. 2005). The increase in bone Mn concentration during the 21 years, and the higher concentrations as compared to results reported by others indicates that the changes in Mn in the burned bones may possibly be diagenetic, for example that Mn is transported into the bones from the groundwater.
The measured concentrations of Fe are not so far from values of Fe concentrations in pig bone reported elsewhere (34-290 µg/g) (Duan et al. 2013; de Vernejoul et al. 1984), but the increase in the Fe concentration in the bones after 20 years of deposit time, still indicates that a transport of Fe into the bones has taken place. Fe and Mn contamination is reduced by mechanical cleaning as well as Al (Skytte and Rasmussen 2013), but some Fe and Mn still remain in the bone structure. The lower concentrations of Mn and Fe in the soil from the pit (even though not lower for Fe) than in the topsoil from the background, might be caused by a washout and transport of Mn and Fe from the soil into the burned bone by the groundwater.
The Hg and Pb concentrations measured in the pig bone are all under the limit of detection. It is theoretically possible that a modern pig could be exposed to Hg and Pb through its food and via vaccines, but since EU legislation demands very low concentrations of the heavy metals in foodstuff, and since the Danish National Food Agency has monitored heavy metals in food for years, concentrations of Pb and Hg below the limit of quantification are expected (Bak et al., 1997; EC 2006). Mercury in the bones might have evaporated during the burning of the pig. The Hg and Pb concentrations measured in soil in this study are comparable with reported average values of the heavy metals in Danish topsoil (Hg: circa 60 ng/g, Pb: circa 12.2µg/g (Bak et al. 1997). The low concentrations of Pb in soil sample AL-10 and AL-11 are probably due to a decrease in concentration with depth. Since the values of Hg and Pb in the bones and in the soil are reliable, and no increase in Hg and in Pb from 1993 to 2013 was observed, Hg and Pb do not seem to be affected by diagenesis.
None of the results in this studyindicate that Ca is affected by diagenesis.The Ca concentrations measured in the study are very similar to the concentration of 37% Ca in animal bone ash, including pig bone ash (Field 2000), and the Ca concentrations in the bones do not change in 20 years of burial.
The measured Sr levels in the pig bones are higher than the Sr concentration of 81.8μg/g previously reported in pig bone mineral (Pagano et al. 2007). This difference might be explained by the provenance of the pig. In this study there is no indication of a transport of Sr into the bone. Strontium does not seem to be affected by diagenesis.
The Ba concentrations in the bones are higher in 2013 than in 1993 and Ba seems to be transported into the bone. Values of Ba in pig bone have not been reported by others however values of 42-87 μg/g have been measured in cow bone (Jaworowski et al. 1985). These values are not far from the values measured in this study.
Conclusion
This study shows how Ba, Ca, Al, Mn, Fe, Hg and Pb are exchanged between the soil and burned, and buried bone. Unfortunately the study only includes six bone samples, all burned and all from the same pig. A larger study including more pigs, more burial sites and a longer deposition period, would provide us significantly more information about diagenesis. However, the results of this study do provide some important information about diagenesis in burned bone.
The results show that Al, Mn, Fe and Ba in the burned bones seem to be affected by diagenesis in over the 20 years, with the effect expected to increase as burial time increases. Aluminium is probably a result of clay contamination from the soil, some of which can be removed during the sample preparation. Manganese and Fe are likely to be transported with groundwater into the bones. Like Mn and Fe, Ba is also transported into the bones. No exchange between the bones and the soil was observed for Hg, Pb, Sr or Ca. The heavy metals in the surrounding soil do not seem to effect the concentrations of Hg and Pb in the bones. Calcium concentrations do not change over the 20 year period, and the observed Ca concentrations in the bones are comparable to values reported by others. Even though observed Sr concentrations in this study are higher than concentrations reported by others, Sr does not seem to be affected by diagenesis either. It is uncertain if the results reported here are valid for unburned bones as well burned bones since the burning process changes the structure and chemical composition of the bone. A similar study of unburned bone could perhaps clarify this.
Competing Interests
The author declares to have no competing interests.
Acknowledgements
I would like to thank Archaeologist and Assistant Curator of Odense Bys Museer Morgens Bo Henriksen for providing the samples. Associate Professor at University of Southern Denmark Kaare Lund Rasmussen and Post Doc Lilian Skytte from the CHART group at University of Southern Denmark for providing the instrumentation, for support and for proof-reading of the manuscript. Anthropologist Lise Harvig from University of Copenhagen for anthropological support. The VELUX Foundation is gratefully acknowledged for the financial support of the project.
About the Author:
Email: lauritsen@sdu.dk
Bibliography
BAK, J., JENSEN, J., LARSEN, M. M., PRITZL, G. and SCOTT-FORDSMAND, J. 1997. A heavy metal monitoring-programme in Denmark. The Science of the Total Environment, 207, 179-186.
CUCINA, A., TIESLER, V., SIERRA SOSA, T. and NEFF, H. 2011. Trace-element evidence for foreigners at a Maya port in Northern Yucatan. Journal of Archaeological Science, 38, 1878-1885.
DE VERNEJOUL, M. C., POINTILLART, A., CYWINER GOLENZER, C., MORIEUX, C., BIELAKOFF, J., MODROWSKI, D. and MIRAVET, L. 1984. Effects of Iron Overload on Bone Remodeling in Pigs. AJP, 116, 377-384.
DUAN, Q. W., LI, J. T., GONG, L. M., WU, H. and ZHANG, L. Y. 2013. Effects of graded levels of montmorillonite on performance, hematological parameters and bone mineralization in weaned pigs. Asian-Australas J Anim Sci, 26, 1614-21.
EC. 2006. The European Commission Regulation: setting maximum levels for certain contaminants in foodstuffs [Online]. Available: [http://eur-lex.europa.eu/LexUriServ/LexUriServ.do?uri=CONSLEG:2006R1881:20100701:EN:PDF].
EMA. 2014 The European Medicines Agency: SUMMARY OF PRODUCT CHARACTERISTICS: Gripovac 3 [Online]. Available: [http://ec.europa.eu/health/documents/community-register/2014/20141204130091/anx_130091_en.pdf].
EZZO, J. A. 1992. A Test of Diet Versus Diagenesis at Ventana Cave, Arizona. Journal of Archaeological Science, 19, 23-37.
FIELD, R. A. 2000. Mini review. Ash and calcium as measures of bone in meat and bone mixtures. Meat Science, 55, 255-26.
GEIER, D. A., SYKES, L. K. and GEIER, M. R. 2007. A Review of Thimerosal (Merthiolate) and its Ethylmercury Breakdown Product: Specific Historical Considerations Regarding Safety and Effectiveness. Journal of toxicology and environmental health. Part B 10, 575-596.
HEDGES, R. E. M. 2002. Bone Diagenesis: An Overview of Processes. Archaeometry, 44, 319–328.
HENRIKSEN, M. B. 1993. Et ligbrændingsforsøg på Hollufgård.-Hvad kan det fortælle om jernalderens brandgrave? Fynske Minder, 99-116.
JAWOROWSKI, Z., BARBALAT, F. and BLAIN, C. 1985. Heavy metals in human and animal bones from ancient and contemporary France. Science of the total environment, 43, 103-126.
LÓPEZ-GONZÁLEZ, F., GRANDAL-D'ANGLADE, A. and VIDAL-ROMANÍ, J. R. 2006. Deciphering bone depositional sequences in caves through the study of manganese coatings. Journal of Archaeological Science, 33, 707-717.
PAGANO, A. R., YASUDA, K., RONEKER, K. R., CRENSHAW, T. D. and LEI, X. G. 2007. Supplemental Escherichia coli Phytase and Strontium Enhance Bone Strength of Young Pigs Fed a Phosphorus-Adequate Diet. The Journal of Nutrition, 1795-1801.
RASMUSSEN, K. L., BOLDSEN, J. L., KRISTENSEN, H. K., SKYTTE, L., HANSEN, K. L., MØLHOLM, L., GROOTES, P. M., NADEAU, M.-J. and FLÖCHE ERIKSEN, K. M. 2008. Mercury levels in Danish Medieval human bones. Journal of Archaeological Science, 35, 2295-2306.
RASMUSSEN, K. L., SKYTTE, L., PILEKÆR, C., LAURITSEN, A., BOLDSEN, J. L., LETH, P. M. and THOMSEN, P. O. 2013a. The distribution of mercury and other trace elements in the bones of two human individuals from medieval Denmark – the chemical life history hypothesis. Heritage Science, 1, 1-13.
RASMUSSEN, K. L., SKYTTE, L., RAMSEYE, N. and BOLDSEN, J. L. 2013f.Mercury in soil surrounding medieval human skeletons. Heritage Science, 1, 1-10.
RAUCH, J. N. and PACYNA, J. M. 2009. Earth's global Ag, Al, Cr, Cu, Fe, Ni, Pb, and Zn cycles. Global Biogeochemical Cycles, 23, 1-16.
SCHUTKOWSKI, H. and HERMANN, B. 1999. Diet, Status, Decomposition at Weingarten: Trace Element and Isotope Analyses on Early Mediaeval Skeletal Material. Journal of Archaeological Science, 26, 675-685.
SEDMAN, A. B., ALFREY, A. C., MILLER, N. L. and GOODMAN, W. G. 1987. Tissue and cellular basis for impaired bone formation in aluminum-related osteomalacia in the pig. J Clin Invest, 79, 86-92.
SKINNER, H. C. W. 2005. Mineralogy of bone. In: SELINUS, O. (ed.) Essentials of Medical Geology. Amsterdam: Elsevier Inc.
SKYTTE, L. and RASMUSSEN, K. L. 2013. Sampling strategy and analysis of trace element concentrations by inductively coupled plasma mass spectrometry on medieval human bones--the concept of chemical life history. Rapid Commun Mass Spectrom, 27, 1591-9.
SNOECK, C., LEE-THORP, J. A. and SCHULTING, R. J. 2014. From bone to ash: Compositional and structural changes in burned modern and archaeological bone. Palaeogeography, Palaeoclimatology, Palaeoecology, 416, 55-68.
SNOECK, C. and SCHULTING, R. J. 2013. Fire and Bone: An Experimental Study of Cremation. Experimental Archaeology.
SNYDER, W. S., COOK, M. J., NASSET, E. S., KARHAUSEN, L. R., HOWELLS, G. P. and TIPTON, I. H. 1975. ICRP Publication 23: Reference Man: Anatomical, Physiological and Metabolic Characteristics, Oxford, Elsevier.
STINER, M. C., KUHN, S. L., WEINER, S. and BAR-YOSEF, O. 1995. Differential Burning, Recrystallization, and Fragmentation of Archaeological Bone. Journal of Archaeological Science, 22, 223–237.
TAO, X., XU, Z. R. and WANG, Y. Z. 2005. Effect of Excessive Dietary Fluoride on Nutrient Digestibility and Retention of Iron, Copper, Zinc, and Manganese in Growing Pigs. Biological Trace Element Research, 107, 141-151.
VAUGHAN, J. 1975. The Physiology of Bone, Oxford, Clarendon.
WILSON, L. and POLLARD, M. 2002. Here Today, Gone Tomorrow? Integrated Experimentation and Geochemical Modeling in Studies of Archaeological Diagenetic Change. Acc. Chem. Res., 35, 644-651.
ZAZZO, A. and SALIÈGE, J. F. 2011. Radiocarbon dating of biological apatites: A review. Palaeogeography, Palaeoclimatology, Palaeoecology, 310, 52-61.