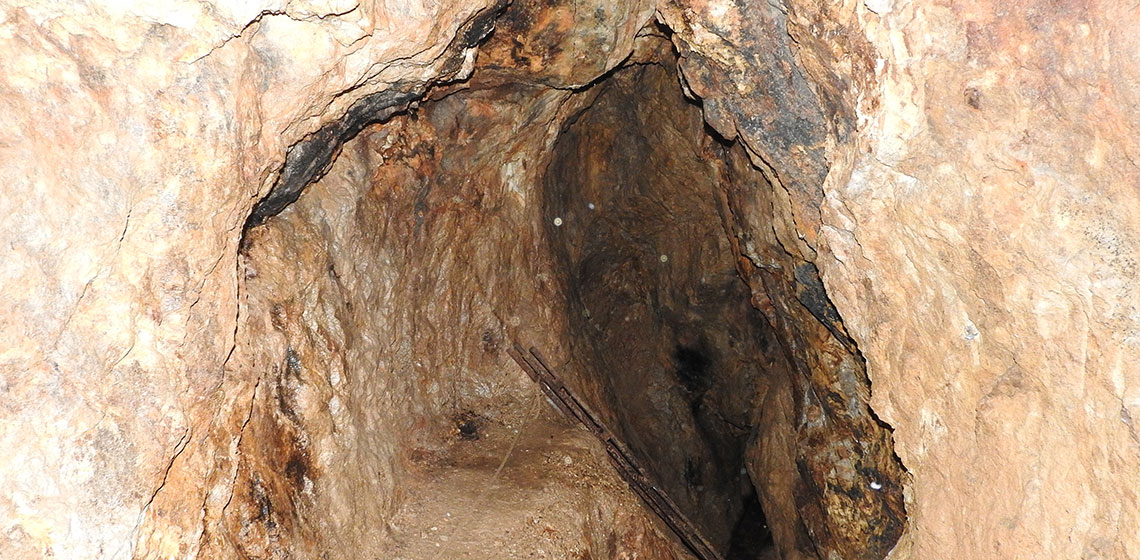
The article is focused on the creation of a protocol for the analytical characterization of fire-setting in different types of rock. A set of experiments of heating and cooling have been carried out on different kind of rocks under various conditions and durations in order to record changes in the structures and composition of the samples. This study was set to support the solution of issues in the case of ambiguous identification between structures due to natural events in the rock and artificial exploitation.
Preview
In the research field of mining archaeology, especially when seeking and assessing ancient exploitation areas in territories that have not yet been extensively studied, or that are not studied at all, several interpretative problems may arise, requiring procedures that are not ascribable to the classical-approach domain. The pioneering study of insufficiently known areas can be faced by the collection of information and indications that comes from disparate sources. Some types of archaeological evidence, such as the finding of metal artefacts or slag fragments, presence of workshops, or mining and metallurgic tools and equipment, can fuel interest in the study of territories that are currently little known from the point of view of the related mining or caving activity. Some clues come as well from the study of historical literature, from the study of toponyms, and from folkloric tales belonging to the background knowledge of communities that often furnish geographical indications of mining areas that are otherwise totally lost. On a larger scale, the landscape archaeology and the geomorphology may come in handy in the identification of mining areas, when for example, the presence of open cast mines, trenches, dump heaps, slag heaps, modification of vegetation, and other macro-effects of exploitation can give fundamental indications for the localization of a mine or a quarry. Often, the identification of peculiar structures in the rocks, in the micro-scale, can also give important information. Some of those special structures in the rock, traditionally and commonly interpreted as natural bizarre, explainable as weathering or structures with particular geologic genesis, may actually hide traces of ancient exploitation. That is why a fire-setting characterization and evaluation through quantifiable characteristics has emerged as mandatory, especially in the field surveys in such of problematic areas. The unequivocal recognition of mining exploitation traces is usually left to the experience of the surveyor, but once no scope for doubt has to be left, it is necessary to search for features that are scientifically quantifiable. This issue has been approached through an experimental reproduction of fire effect on different types of rocks (limestone, sandstone and granite) under different conditions (of duration, temperature and cooling means - with water, vinegar or under room temperature) in order to obtain a characterization of the fire effect on different typology of rock. The samples of rock have then been observed, measured and analysed in order to identify unequivocal features (changes in elastic modulus, strength, presence of defects, modification in the mineral phases, identification of high temperature transitions) that can be used to unravel difficulties in the distinction between artificial exploitation of the rock and natural effects.
Introduction
Fire-setting, the technique of breaking the rock, by softening it through the application of a wood fire on its surface, is the most ancient and the most long-lasting type of raw material extraction from the Neolithic period (for example Aibunar in Bulgaria or Kleinkems in Germany around 4000 BC) and used into post-industrial times (until the 20th century). There are several indications of fire-setting in textual documents such as the Bible, in both Jeremiah (628 BC) and the Book of Job (around 400 BC), where the fire-setting is described, as a paradigm of the strength of God’s word (Willies and Weisgerber, 2000). In Agatarchides scripts (II century BC) there is a very detailed description of fire-setting in the Egyptian gold mines of the Red Sea area (Burstein, 1990, pp.65-66). In Livy’s and Pliny’s texts reporting the tale of Hannibal passing the Alps through the application of fire-setting quenched with vinegar - infuso aceto - to create tunnels of passage (Willies and Weisgerber, 2000). In Pliny’s Naturalis Historia, another interesting description is reported regarding the gold mining activity in the North-West of Spain, where fire-setting aided by vinegar was indicated as the operation used to weaken the siliceous rock (Craddock, 1992). In more recent times, fire-setting is described in detail by Agricola (1556) together with very vivid illustrations.
The reason why the fire is used to break blocks from massive rocks is to be found in the physical results of an abrupt change of temperature in a heterogeneous medium like a rock. In fact, a different coefficient of expansion of different minerals will cause inner stress that will lead to micro-cracks. The thermal gradient between the superficial level and the internal bulk will cause a differential expansion of layers of rock following its profile from outside inward. The breakage of the rock can be further accelerated by the rapid cooling of the rock surface with water or other means of quick chilling (causing again abrupt thermal gradients in the rock that cause a final deterioration of the original structure).
Fire-setting was performed mostly with dry wood, in order to reach high temperatures with a minimal production of fumes which could cause danger in an underground environment. The temperature of a fire, which can be mainly considered without air draft, has to be positioned in the range between 500°-1000°C (Goudie, et al., 1992).
In this range of temperature, the rock is affected by various transformations, such as differential expansion due to varying thermal behaviours of different minerals or the release of water content of the rock pores but also of water of crystallization, chemically bound, coming from many minerals constituent rock. Also, the differences of expansion of crystals along the different crystallographic axes (except for isotropic minerals in the cubic system) and the different expansion under temperature gradients (Willies and Weisgerber, 2000) from the heated surface, decreasing towards the inside of the rock wall attack the rock structure. Additionally, the release of gases, like carbon monoxide and dioxide, in the case of calcination of calcium carbonate at about 900-1000°C, is a phenomenon that occur during fire-setting. The final quenching causes a second abrupt change of thermal condition of the system, causing a final thermal shock. The changes of phase of minerals from room temperature to high temperature stable phases, persist in the rock once it is quenched with water or vinegar. Heating or cooling rates of 2°C or more per minute cause spontaneous cracks in rocks such as granites, schists and diabases (Saiang, 2011). The ejection of fragments - “spalling” - can also be promoted, not only by the thermal gradient but also by a low thermal diffusivity, texture and anisotropy, that is a variation of the thermal capacity in different directions (Goudie, et al., 1992), a high moisture content (and relative steam pressure), a low porosity and the previous application of a compressive stress (Nordlund, et.al., 2014) that could be predominant for instance in metamorphic rocks and lithostratigraphy coming from tectonic processes like folding. The fire effects on stones include varied typology of damage or transformations that, from the macroscopic result they have on the rock, can be classified as breakage, spalling, crenulating, crazing, potlidding, microfracturing, pitting, bubbling, bloating, smudging, discoloration, altered hydration and weight and density loss (Deal, 2012).
Each of these kinds of transformations can be evidenced by specific analytical techniques. Mechanical tests are used generally because all these transformations determine a variation in the mechanical properties of the rock such as strength and stiffness that is the capability of a litotype to oppose elastic deformation (Saiang, 2011).
The present article describes research composed of experimental reproductions and analytical characterization, conducted in order to produce a protocol aimed at analytically distinguishing ancient fire-set rock. What complicates the creation of this scientific protocol is that each different kind of rock shows basically a different kind of effect after the heating at high temperature. These considerations allowed us to indicate which will be the most suitable analytical procedures, up to the task of distinguishing between a weathered sample of rock and a fire-set sample.
If the method of fire-setting in ancient mining is quite well known and profusely experimented from the point of view of duration, fuel consumption and methods of application, then a systematic study about the distinction between natural weathered rock and heated rock and the identification of un-ambiguous features of identification is here faced through experimental and analytical methods.
Several interesting trials in experimental archaeology have been executed in past times and were especially conducted in order to verify the actual feasibility of fire use for the opening of galleries, and in order to understand the amount of wood needed (and the consequent impact on the environment), the duration of fire treatment and the residual materials left (Picking and Timberlake, 1988; Crew, 1990; Timberlake, 1990a and 1990b; Craddock, 1992; Py and Ancel, 2006; also Per Storemyr and Florian Tereygeol with their online documentaries of experiments, just to cite some examples).
The scientific question
What is commonly enounced as very distinctive traits of fire-setting in the rock is the very smooth surface, the rounded and sinuous profiles, roofs blackened by soot and the associated layers of ashes and charcoal mixed to rock flakes on the ground. Unfortunately, the experience collected in different areas of the world with differences in climate, vegetation and geology, allows us to say that often the structures are less clear and much less tell-tale. First of all, the dimension of the mining structures and the degree of opening to the outside can cause the dispersion of the lighter and finely particulate material, such as coal and ash. From experience gained in the field it is possible to say that the more ancient the structure, the more likely it is that the development is superficial, with galleries still in place. In many cases, galleries may have collapsed, leaving an altered and confused landscape. Many times, it is heavy wind, washout or the coverage of the original walk-planes with soil and vegetation that causes the unease of interpretation. That means that in particular environmental situations, such as when the weathering is extreme (wind erosion, water erosion, karsts effects) or in the case where the geology of the rock is itself particular (faces of contact of carbonatic and silicatic rock that can cause differential erosion, or in the case of volcanism generating lava tubes for example), there can be false interpretations of landscape. It is the case, for example, of grottos that are commonly interpreted as karsts by geologists, but that can show in some special cases, instead of natural shapes of solubilization, the typical concave shape of fire-set rock and a sort of patination/vitrification of the surface (different from the redeposition of fine layers of secondary limestone), or other artificial shapes. Therefore, in field surveys aimed at the search of evidence of ancient mining activities, the experience of the prospectors is a fundamental factor in the identification of structures of fire-setting in the rock, but in order as well to communicate and show the result to the public, analytical data can be sometimes easier to transmit, more clearly understandable and they would eliminate any doubts in the scientific discussion.
During the field surveys conducted in 2014, 2015 and 2016, in Portugal, Italy and Morocco during the post-doc research program financed by the Blanceflour Foundation and hosted by the Deutsches Bergbau Museum, several natural grottos and so-called ‘natural’ rock shelters have been checked directly, and in some cases the interpretation produced contradictory results. In these cases, finding an unequivocal factor that allowed the distinction between natural and fired rocks was evaluated as mandatory (See Figure 1).
Methodology
The elastic modulus as an indicator of fire operations on rocks
From a well-established scientific literature on structural engineering concerning the analysis of buildings affected by fire events (Saiang, 2011; Sanjurjo, et al., 2013; Nordlund, et al., 2014), on geological and ecological studies (Goudy, et al., 1991; Allison and Bristow, 1999; Brotons, et al., 2014), and also archaeological studies (Deal, 2012), many results are reported indicating various detectable changes in the stones. Among the mechanical tests more commonly used stands the measurement of Young’s modulus and Poisson’s Ratio to detect the variation of elasticity. The measurement of elasticity can be applied experimentally to the rock without the necessity of sampling. That is why, in order to respond to the necessity of non-destructive and in situ measurement, it was decided to deepen the study on elastic modulus and its changes in different types of heated rock. The modulus of elasticity is a value, a number that describes the response of a rock under a mechanical stress, and its resistance to being deformed in an elastic way. This feature can be detected using ultrasound apparatus, an ultrasonic pulse velocity testing equipment that measures the sound velocity in a solid material. In the DBM laboratory we chose to use a Lighthouse UMPC instrument, working with Georg Wange, who kindly facilitated the setup of both equipment and measurement.
The tool is composed of a longitudinal wave transducer that sends the wave into the material, and a receiver that acquires the wave and converts it into an electronic signal. The ultrasonic receiver was a digital storage oscilloscope or PicoScope 3224 transient recorder, which converts the received signal from analogue to digital, in connection with the ultrasonic generator USG 40 (Ultrasound impulse generator). The wave test probes are attached to the opposite ends of the measured object.
The travel time measurement, the path of the measurement, and measurement of the speed of the sound, together with the detail of the sample such as the dimensions, the weight, and the density, were considered in the calculation of the elastic modulus. In solid matter, the types of waves were:
- pressure wave/ longitudinal wave
- dilatational wave/ rod wave
- shear wave/ transverse wave
- Rayleigh wave/ surface wave.
It is possible to use the equipment for determining the propagation speeds of elastic waves in stones and concrete in different ways, by positioning the test probes differently.
Reflection measurement (See Figure 2a), very often used in metal testing as a pulse-echo method, can only be used on sampled specimens. By using this positioning, it is possible to evaluate the inhomogeneities (aggregates, cracks), high sound absorption (porosity), and insufficient reflection horizons (uneven back wall).
The through-transmission or transmission measurement (See Figure 2b) is applied frequently for testing techniques and it is used to determine the longitudinal wave speed, evenness and homogeneity, damping behaviour and acoustic transmission behaviour of the sample.
The surface measurement (See Figure 2c) is only used for samples which are only accessible from one side, or to determine weathering depths. This geometry was used for the in situ measurements. Besides the pressure wave, the speed of the transversal and Rayleigh waves (VS and VR) can also be determined.
The ultrasonic transit time measurement with excitation pulse is dependent on the quality of density and heterogeneity of the rock itself, and on the quantity of plans of discontinuity that decelerate the velocity of the sound wave. The damping is relative to the elastic property of the material. The more the rock is unaffected by microfractures, the faster the speed of the wave in the material. The voids are also an important factor that determine the drop of velocity, depending on how they are refilled: the water refilling of a cavity will cause a faster transduction of the sound wave, as in water the sound is three times faster than in the air. A dry void will cause a deceleration of the wave propagation.
In using the mobile version of the apparatus, the elastic modulus was defined as dynamic elastic modulus in order to distinguish it from the static one, acquired on standardized sampled specimens. The two methods provided different results, sometimes divergent for inelastic rocks, due to the different amplitude of the wave used in the two different methods (Brotons, et al., 2014). These discrepancies were solved by considering the results obtained in the in situ trials only as relative different propagation velocity values coming from fired and non-fired rocks. In the outdoor environment, the weathering effect and the resulting decrease of competence of the rock has to be considered as one of the elements that causes a drop of elastic modulus (Goudie, et al., 1992). By collecting measurements in rocks unaffected by fire, in order to obtain a characterization of unfired, weathered rock, is possible to figure a baseline for the evaluation of the response given by fire-set rocks.
XRD and Raman spectroscopy for high temperature mineral phases and optical microscope for the changes of structure and mineralogy
At the end of the ultrasound measurements, a set of characterization analyses have been done with the intent to understand the changes in the samples that actually cause the modification of rock elasticity. X-ray diffraction (XRD) on powder samples has been accomplished for the identification of mineral phases that are crystalline or sub-crystalline, with a discrete X-ray pattern. The detection of the amorphous fraction of the sample is then unclear because of a lack of long range order and consequently sharp peaks. The instrumental limit of detection (LOD – minimum presence of a compound that can be detected) and limit of quantification (LOQ – minimum amount of a compound that can be quantified) of the XRD will not allow detection of the phases that are present as minor components. The detection of high temperature transitions is not assured in all cases because they often cause slight changes of mineralogical structures that are not always easily detected. These transitions can result in weak shifts or deformation of the normal diffraction peaks.
After a semi-automatic identification of XRD peaks found in the powdered samples, a manual re-evaluation of peaks on the spectra was performed to identify the beginning of phase transitions in the experimental temperature range. Raman Spectroscopy can detect any changes in the vibrational and rotational modes under laser excitation, between the room temperature stable phases and the transition to high temperature phases. Slight changes in the structure of a compound can give identifiable changes in the peak of Raman spectra (Bishambhar and Hari, 1949; Bates and Quist, 1972; Kazuaki, 1975; Barker and Robinson, 1984, Heaney and Veblen, 1991; Mahfujul, 2011). That is why the use of these combined techniques can provide comprehensive information about the high temperature phases.
With the aim of detecting the grade of mechanical stress in the rock, investigation of thin sections of material, with an optical microscope, can give important indications on the grade of fracture and loss or changing of mineral phases.
The research presented here is based on the four techniques discussed above, but Raman elaboration is currently still in progress. In this article the major results of XRD and optical microscope are summarized and an introduction to the Raman spectroscopical elaboration will be presented shortly.
Experiments and results
Experimental methods
A series of experiments was planned and executed between November 2016 and January 2017 on blocks of schleriter sandstone, wurzeldorfer quarzite, calcite and granite. The experimental set was composed of different steps and articulated in three different practical approaches, as reported below:
First phase of measurements – natural fire and natural environment
A first experiment of reproducing fire-setting in a small scale was executed by firing cubes of 5 cm per side, of different kind of rocks and under different cycles of fire- cooling events (See Figure 3).
For each category of blocks, 6 samples were fired in a hole dug in the ground. A fire made of dry wood was set in the centre of the hole, while the blocks were posed around and partially covered by soil on the top, in order to expose mainly one side to the direct fire. The temperature of the fire was measured with a thermocouple and maintained at a maximum of about 600°C for the first 1.5 hours. After this time, two blocks per type of rock were removed from the fire and one cooled under air and one with water (by dipping the block in a bowl). The residual blocks were fired for another 1.5 hours at an increased temperature of about 900°C. After this step, again two blocks per type of rock were extracted from the fire and cooled down, one under environmental conditions and one quenched with water, as before. The final two blocks per type of rock were cooked for an additional 1.5 hours at the same temperature (total 4.5 hours). At least three control blocks were preserved as they are, to evaluate the ultrasound technique and the response given by a non-fired rock.
After this experimental phase, all the blocks were dried in the electric oven by 56°C, a temperature which has no effects on the rock structure, to eliminate the humidity that permeated the rock. At the end of this preparatory phase, all the blocks were measured by means of the ultrasound equipment, using a fixed geometry, in order to have the maximum evaluation of the velocity of the sound wave in the rock. All the values of the elastic moduli were then plotted to clearly display the differences and to evaluate the validity of the method. The plots were divided by type of rock and, for each type of rock, 4 non-heated blocks were measured in two different directions: one parallel to the rock natural stratification, if any, and the other in the orthogonal direction, to detect any changes due to the nature of the rock itself. After this preliminary set of measurement, the heated blocks were measured along a direction orthogonal to the fired surface, the first value taken at about one cm from it, one at the centre and one at about 4 cm away from the fired surface (in the graphs indicated with 1, 2, 3), to detect differences in the elastic modulus along the profile of thermal gradient. A first plot is always reported, showing the results obtained in the measurement of elastic modulus in the natural non-fired rocks. As can be easily seen, there is a variation in the field of values that is due to the heterogeneity of the rock. What is fundamental to note is that the natural range of variation is always narrower than the range of variation that is measured in the fired rocks. So, this simple test allowed us to say that the method is good in the distinction of fired and non-fired rocks, as the variation field is much bigger when considering fired and non-fired rocks together.
After the first 1.5 hours at 500-600° C, the rock had an elasticity that is comparable with the natural rock, even if a small decrease of elasticity is already visible and appreciable. In the samples that have been fired longer and under higher temperatures, and especially on the samples that have been cooled down with water, the decline of elasticity can reach lower values. Long-heated granite samples can see a decline of elasticity of up to 60KN/mm2.
The four schleriter sandstone reference blocks always show a lower value of elastic modulus in the direction transverse to the texture. That is explained by the presence of stratification of layers in the sandstones and the relative discontinuities at the interfaces (See Figure 4).
The variation of elastic modulus was, in the unheated schlerite, due to the heterogeneities of the rock, in a range between 16.4 and 20.2 kN/mm2.
After thermic treatment, the decrease of elastic modulus was coherent with the timing and increase of temperature. Quenching with water created an abrupt drop of values for the blocks heated for 3 and 4.5 hours (See Figure 5).
On the four Wurzeldorfer quartzite samples, the distribution of the reference values is more random, probably due to the major degree of compactness. The variation range is about 10 kN/ mm2 among 11-21 kN/mm2 (See Figure 6).
In the treated samples, the values of the elastic modulus of the blocks treated for 3 and 4.5 hours show a drop of value, especially for the blocks quenched with water (See Figure 7).
The case of the granite rock is the most peculiar, showing a high range of variability in the reference samples and high values of elasticity. There are only two references in this category because of a lower availability of this material (See Figure 8).
After the thermal treatment, the values are more grouped together in three areas of the graph, evidencing very similar values for the blocks heated for 1.5 hours and quenched with water and the blocks heated for 3 hours not quenched, while the blocks treated for 3 hours and then cooled with water and the two blocks treated for 4.5 hours show comparable values (See Figure 9).
In the case of the granite, it is possible to state that quenching with water saved 1.5 hours of fire-setting, by giving a similar value of elasticity for the block heated for 3 hours and the two blocks heated for 4.5 hours.
Calcite blocks show a peculiar trend where, in almost all cases, there is a minimum in the middle measurement. This trend appears in a lesser extent in the other kind of rocks, where the median value appears to be a minimum or in some cases a maximum. That could be caused by the fact that the propagation of the wave in the central part occurs in a different way than in the areas at the borders, where the wave is partially dispersed out of the block. Other than this characteristic of the measurements, calcite is an elastic rock with an ample range of variation (See Figure 10).
After the treatment the blocks show an abrupt decrease of elastic modulus, that starts to appear after one and a half hours with water quenching. Again, the sample quenched with water after three hours of treatment have values that are analogous to the samples treated for 4.5 hours (See Figure 11).
This first set of experiments had the function of evaluating the methodology. Three other types of experimental approaches have been attempted in order to finally validate the procedure.
Second step of experiments – electric oven, controlled conditions and use of vinegar and water for quenching
A second set of experiments was executed to evaluate the effects of using vinegar instead of water to cool down the samples of rock. The use of vinegar after fire-setting was tested as it is described in ancient sources such as Pliny and Agricola, especially in connection with calcite rock (See Figure 12).
Theoretically pure water is the best liquid to cool down a system, because the chemical bonds of the water molecule are more energetic than, for example, those in acetic acid which is a more volatile liquid requiring a smaller amount of energy to pass from liquid to gas (1 kg of water absorbs 336 kJ by heating from 20°C to boiling at 100°C whereas the same mass of acetic acid only absorbs 163 kJ - https://www.engineeringtoolbox.com/specific-heat-fluids-d_151.html). This is why this historical source was used as a starting point for this set of experiments, triggered by the questions of why and how could vinegar help the fire-setting procedures, particularly of calcite rock?
This experiment is composed of several phases: the blocks were placed in porcelain bowls filled with sand, in order to evaluate the heating effects on one side of the blocks, thereby reproducing as much as possible the situation of fire-setting (it was not possible to use the same conditions so the use of the electric oven was preferred considering the comparability of the results). The same encasing in fine sand, exposing only one face of the cubic blocks, was described as experimental setting in Allison and Bristow (1999), to simulate the natural environment. Fine sand succeeds the best in the recreation of the diffusion pattern of thermal energy on a large exposed surface (Allison and Bristow 1999). The first step of heating for 1.5 hours was skipped as, from the evidences arisen in the first experimental set, it brings less information then the following two steps.
For about 3 hours the temperature in the oven was raised to 500° C, then one block was removed from the sand and cooled down with water. The second block was cooled with vinegar (a 26% solution of acetic acid in water). The residual two blocks have been heated in a second bowl for 1.5 hours at 1000°C on one side only (as explained previously) then one block was cooled with water and one with vinegar. The following plots shows the results obtained for granite, calcite and the two types of quartzite.
In the case of calcite it was impossible to cool the 1000°C block with vinegar because at 500°C the reaction between calcite and vinegar was extremely violent, resulting in an intense effervescency.
So, after 1.5 hours the blocks heated to less than 1000°C were cooled, under environmental conditions, and the other under water (See Figure 13).
The other blocks followed the normal procedure, as described previously.
Calcite | The values of elastic modulus could not be evaluated completely because of the explosion of the block which was quenched with vinegar. Surprisingly, what appears is that for the residual part of the block quenched with vinegar at 500°C, the values are lower than the water quenching, but not showing an extreme change in the macroscopic aspect. Hypothetically, the very energetic bonds of the water molecule provide a better cooling effect on the calcite; in fact the elastic module values are lower in the case of blocks cooled with water instead of vinegar. From the direct observation, the blocks are completely decomposed after the cooling down with vinegar (See Figure 14). From a direct observation of the effects of vinegar on the rock, the effervescency testifies the evolution of carbon dioxide caused by the reaction between the CaCO3 and acetic acid, that is faster and heavier (See Figure 15). when vinegar is used in place of water. The chemical reaction and the production of gas is the cause of the disaggregation of the rock in this case, probably added to a mechanical effect of the gas pressure of the developed CO2 that in some cases can cause the ejection of real projectiles. So, we can conclude that in calcite the vinegar chemically decomposes the rock, but the cooling gradient is less steep than with water. As it will be explained by the plots of the other types of rocks, the function of vinegar in fire-setting, works best for carbonatic rock, which seems to match well with the ancient textual sources. |
Granite | In the case of granite, as expected, there was an increase of temperature of the thermal treatment and some slight evidence of a change of cooling effects from the use of water and vinegar at 500°C (See Figure 16). |
Schleriter sandstone | In the case of schlerite, the elastic modulus decreases, as expected, with the increase of temperature of thermal treatment but there are no evident changes of cooling effects between the use of water and vinegar; indeed, it shows a slight increase in the elasticity of the rock at 500°C and a small decrease for the block treated at 1000°C (See Figure 17). |
Wurzeldorfer quartzite | A similar pattern is shown with the Wurzeldorfer quartzite. The vinegar does not work better then water as a coolant. It was also noted that the blocks heated above 900°C, as in the first experiment, show a decrease in cooling that looked more affected by mechanical stresses after the use of water to cool it of elasticity of more than 4 kN/mm2 (See Figure 18). |
Third experiment – portable ultrasound equipment in the field
After this preliminary approach in the laboratory, a field mission was undertaken with the aim of validating the usability of the method with a portable ultrasound equipment. The change of geometry from the fixed setting and the use of the portable gun, causes a deterioration of the quality of the measurement, as the wave is measured in a geometry where the detector is not in the longitudinal direction of the transmitter but it is instead positioned on the same side as the transmitter (See Figures 19 and 20). Despite this limitation, it was evaluated in the lab that the variation of velocity of the soundwave from the fired and non-fired rock can be appreciated, allowing the use of the method in the field. By giving the software values of density and penetration of the wave in the rock (that are somewhat arbitrary) it was possible to extrapolate a value of elastic modulus. The protocol was tested in December 2016 in two ancient mines: Sankt Ulrich-Birkenberg and Kleinkems in the Baden region. Saint Ulrich mine is an exploitation in gneiss rock opened in the medieval period for mining Tetrahedrite (a complex mineral of the fahlore group, containing copper, antimony and sulphur) while the Kleinkems mine was exploited during the Neolithic period for flint. Both mines have very visible, identified fire-setting areas that can be used to check the validity of the ultrasound method.
By using the portable method in the Kleinkems flint mine, a high decrease in the velocity of sound was detected in the areas where fire-setting was used, in comparison with the natural rock. The value of elastic modulus for fire-set rock is 2.8 kN/mm2 instead of 17.8 kN/mm2 of natural rock in the area. Relative running times of the measurement of respectively 46.9 µs and 5.8 µs. This result is in agreement with the experimental results obtained in the laboratory. This mine is open cast, where natural unexploited rocks are easily distinguished.
In the area of Sankt Ulrich, the fire-setting niches in the main gallery of exploitation gave widely varying values of time required by the sound wave to reach the detector, in some cases opposite to the expected trend. In the fire-setting rocks it was in some cases faster, thus giving an idea of more elastic rocks, while the areas which were exploited by the means of mining tools gave mainly higher values in seconds. The values of mean running time are of about 11.25 µs and an elastic modulus of 51 kN/mm2 for fire-set rock, in comparison with the slightly higher mean value of running time in non fire-set rock of about 12.62 µs and a mean elastic module of about 30.2 kN/mm2. This opened new questions: why were the results on gneiss sometimes opposite to the trends in calcite? First of all, it is important to state that a similar result of stable values or smooth variations between fire-set and non fire-set stones was observed in Schleriter sandstone and Wurzeldorfer too. Beside this preamble, a working hypothesis was formulated: in gneiss, the percolation of ground water causes a soaking of the rock and, since in the water the sound propagation is faster, the time required for the wave to reach the detector may be shorter. In fact, in the fire-set rock the fractures and microfractures can be refilled by water creating a “sponge system” that is actually more elastic than the normal natural rock (less fractured so less affected by infiltration). Because the pressure wave velocity in water is several times higher than in air, the velocity generally increases in saturated hard rocks (Lama et al., 1978). Nevertheless, it must be considered that the pressure wave velocity in water is lower than that of the mineral structure (Eitzenberger, 2012). In the calcite, the hypothesis created is as follows: the kind of mining structure and the difference of rock (denser and less hygroscopic) allow the water to flow in it. This particular question required the opening of a new set of experiments, planned to examine how much the meteoric water in the rock affected the elasticity of it. A final set of measurements with soaked rocks was executed, again in the laboratory, to evaluate how the elastic modulus changes with respect to water content locked in it. For the future development of this research, a possible solution for in situ measurement is the use of shear waves instead of compression P waves, as they allow an evaluation of saturation degree, and a correction of the final result.
Fourth experiment – again in the laboratory to evaluate the water impregnation of the rock and the elastic modulus changes
This final step was executed on the blocks of the first experiment, placed in water overnight. So, the blocks here considered were previously heated for 1.5 hours, 3 hours and 4.5 hours and quenched with water, or cooled in room conditions. In order to minimise ambiguities with the first experiments, the samples were distinguished by the use of a new labelling system: the blocks A are the Schleriter sandstone, B is the Wurzeldorfer, C the calcite, and D the granite, while the number 1 indicated cooling with water and number 2 cooling at room temperature. In these final graphs, the trends are the same as in the first experiment, but the cracks refilled by water give a higher value. The presence of water in the cracks, microcracks and voids caused by the fire-setting were filled by water depending on how permeable the rock was and how much the cracks were open or closed. That could explain why conflicting results were obtained from calcite and gneiss (See Figures 21, 22, 23 and 24).
The graphs give interesting results concerning the trends of elasticity in these rocks, by showing a variable response to the presence of water. The elastic module decreases in relation to the temperature of fire-setting, without being unduly modified by water. That is a positive result in a way, as we can state that the portable protocol can be used in the field where the rocks are obviously impacted by rain and washout. Additionally, it is possible to see that, especially in the case of calcite, the range of variation of the elastic modulus increases extensively, while for the other type of rocks the effect seems less evident. In order to evaluate the power of the method and the problems that can arise in the field because of possible noise of signal, connected to peculiar types of rocks, it is useful to execute a comparison between the results obtained in the first set of experiments and the third (See Figures 25, 26, 27 and 28).
In the Schleriter sandstone and in the granite, the take up of water causes an increase of the values that is clearly visible by comparing the graphs. In calcite, the numerous cracks can, in the samples heated for 3 and 4.5 hours, show similar values of elasticity between the water-saturated samples and the non-water-saturated samples, suggesting that the more numerous the cracks are, especially in the case of open, connected cracks, the more the water is able to drain out. Beside this, the big dimension of the cracks can cause a difficult saturation for capillarity. This could suggest why, in the mine of Sank Ulrich, the ultrasonic device gave higher values of velocity, in comparison with the non fire-set areas. In this mine especially, we have to consider that the measurements have been done in a gallery that is placed at around 15 meters horizontally into the mountain. The galleries are excavated in the area of permeation of ground water, where the pores and cracks in the rock can be estimated as filled by water. In Sankt Ulrich, the gneiss can be defined as soaked. In the case of Kleinkems, the flint mine is not a deep exploitation with galleries, but an open cast mine at the surface of the limestone. In this situation, instead of collecting at the cracks, the water flows, creating enlargements of the cracks and natural porosity. Therefore, the different results obtained from the two mines can be explained by the different conditions of type of rock and types of exploitation. In the case of Saint Ulrich, the differences of values in the fire-set and non-fire-set areas made the differentiation of the two types of zones in the mine apparent. The different responses given by fire-set rocks and the areas exploited by tools or the non-exploited areas of unworked rock allow a calibration of measures on a case-by-case basis, explaining some divergences of results coming from the laboratory and obtained in the field.
Two final comparison graphs have been produced in order to show the final results of the research of rock elasticity (See Figures 29 and 30).
The decrease of elastic modulus in relation to temperature and time is a trend that is appreciable in all the type of rocks studied. What is remarkable is the extreme drop of mechanical quality of calcite and granite, in comparison with the less steep decrease shown by the sandstone and quartzite rocks. This behaviour can be explained by the homogeneous structure and competence of the former rocks and of the dominance of compacted quartz grains in the latter.
This result allows hypothesizing that the fire-setting exploitation of sandstone and quartzite required a higher amount of wood and longer periods of treatment, rendering this application less convenient in terms of supply of fuel. In sandstone, due to the relative softness of the rock, generally mining exploitation was conducted with tools. In quartzite, the high hardness of rock made the using of fire-setting necessary, even if high in labour and time costs. This result fits well with evidence coming from mining archaeology: if the fire-setting of rocks such as calcite, granite, gneiss and quartzite and other stones with more complex mineral composition is common in the ancient quarries and mines, then evidence of exploitation of sandstone with fire-setting is unknown.
The protocol proved to be usable in the case of uncertain identification of a weathered rock or fire-setting areas and it will be utilised in other areas that seem to be much more connected to artificial exploitation than to karstic cavities.
XRD for characterization of samples and for detection of changes in mineralogical phases
At the end of the experiments in both field and laboratory, XRD analyses have been performed on the series of blocks of the first set of experiments, renamed after experiment n.4 (A, B, C and D blocks) and on samples of fire-set and non fire-set rocks of Sankt Ulrich. In order to speed up the analyses, only the samples of reference (controls A0, B0, C0 and D0) and the blocks fired for the maximum extent of time at higher temperatures and quenched with water (A31, B31, C31, D31) were analysed. Beside this set, samples of fire-set and non-fire-set rock from Sankt Ulrich have been analysed, in order to get results from the gneiss. The analyses were executed by Jan Sessing at the XRD (Cu K(α)) of the DBM. Preliminary analyses on calcite with dolomite and quartz phases were done together with Dr. Katrin Hitzler (in that period DBM-Bochum), who noticed a transition to some high-temperature phases such as pigeonite and diopside. The diopside can be generated by the reaction of the dolomite with quartz under increasing temperatures (Lüttge and Metz, 1993), while pigeonite is a clinopyroxene that is stable at temperature over 800°C. Also, an interesting indication was obtained on the quartz peaks, showing an increase of volume and a slight shift that could be interpreted as the passage from the alpha phase of quartz to the beta phase that is stable at temperatures above 573°C and at room pressure. The beta quartz has a volume 0.8% higher than the lower temperature phase.
In the experimental samples, a similar shift was noticed but in the following cases the interpretation was more complex and partially done manually. At a first glance, the references and the fired rocks spectra seemed similar.
Sample A0 is a sandstone composed of quartz, albite, muscovite, clinochlore and anorthoclase and A31 analogously showed the same phases, with the sole absence of the clinochlore. | See Figures 31 and 32 |
Sample B0 is a quartzite composed of quartz and microcline feldspar and so is B31. | See Figures 33 and 34 |
The C0 sample is a limestone composed of calcite, as is C31. | See Figure 35 |
The D0 sample is a granite composed of quartz, albite, muscovite and orthoclase and so is the D31 sample with an additional minor phase of biotite. | See Figures 36 and 37 |
Finally, the gneiss from Sankt Ulrich showed the phases of quartz, albite, muscovite and clinochlore and the fire-set sample likewise confirmed the major phases. | See Figures 38 and 39 |
Only after the post-processing was done manually, with the interpretation of the minor peaks, by zooming the sector of the spectra between position 18 to 50 °2Θ and zooming in the area of the low counts, some minor phases appeared. In sample A31 the peak at position 26° 2Θ has a higher intensity than the references, suggesting a possible transition to the albite phase at high temperature (albite high, De Caro, et al. 2013). The peaks of quartz have an increase of volume and a shift to the right, that could be assigned to the transition to the beta phase. In the case of sample B31, the quartz seems slightly shifted to the right. The overlapping of the peaks of microcline and its high temperature phase orthoclase don’t allow a detection of this possibly present minor phase. In the case of the calcite a minor peak appears at position 26° 2Θ which can identify the presence of a minor phase of CaO that develops at over 900°C for calcination of CaCO3. All the other peaks of CaO are overlapping the peaks of calcite, causing a difficulty in distinction, but two shoulders of the peaks of calcite at positions 58 and 61° 2Θ could be assigned to a minor presence of CaO. In sample D31, again the estimation of the presence of albite high can be determined by a slight increase in volume of the peaks from 22 to 26° 2Θ of the spectrum and a peak at 27° 2Θ. Again, the quartz seems bigger in volume and shifted to the right. In the sample of fire-set rock from Sankt Ulrich, the quartz shows an increase of volume and a shift (to the left this time), while in the area between 22-26° 2Θ and 27-29° 2Θ, the higher intensity of the peaks normally assigned to the albite could be interpreted as the additional minor phase of the high albite.
In conclusion, the fire-setting, due to the limited time of the process, can activate reactions of phase transformation to high temperature phases that can be difficult to detect by the XRD.
Those phase changes, for example the transformation from CaCO3 to CaO with the release of CO2 even if not extensively developed, are enough to cause an inner stress that decomposes the competence of the rock. The same happens in the other types of rock, and together with volume increases, contractions and loss of water, cause the disaggregation of the rock. The difficulties in showing evidence for the minor phases with the XRD led to the decision to enlarge the protocol of study to the use of the micro-Raman spectroscopy. This is currently work in progress in the laboratories of the ISMN-CNR of Rome, with Dr. Tilde De Caro. From a detailed study of specific literature on Raman characterization of the alpha-beta quartz transition, some parameters of spectra interpretation have been extracted and they will be used for the detection of this transition. The reduction or disappearance of peaks during the development of alpha to beta transition could be used with this aim. These preliminary considerations will give direction to the further experiments that will be set for the future.
Polarized Optical Microscope
Thin sections of control samples and experimental samples (fragments coming from the directly heated side), were kindly prepared for observation under the optical microscope by Andreas Ludwig, in charge of the Preparation Laboratory of the DBM. The sections were prepared for the following samples: A0, A31, B0, B31, C0, C31, D0, D31 and fire-set and non fire-set samples from Sankt Ulrich.
The images recorded by Dr. Stephen Merkel have supported the interpretation phase, by using the polarized and crossed polarized mode, to detect and recognize the mineral phases and the reactions occurring. Some changes were recognized in all the fire-set samples, such as darkening and increment of cracks (intra and intergranular, only in the schleriter these are less visible).
In the gneiss from Sankt Ulrich, the mica became yellowish-gold after heating, and the biotite became darker in colour (this generally happens over 800°C). In this case, the cracks in the reference rock are refilled by secondary minerals, while in the heated sample new fresh cracks can be distinguished as they are empty (See Figure 40).
Conclusions
The experimental procedure to replicate the fire-setting effect in rocks, done in experimental archaeology and in the laboratory, was necessary for the development of an analysis protocol to distinguish fire-setting in situ and on samples collected during field work. The procedure is suitable for the resolution of the problem presented. This protocol will be applied to unravel the interpretation of structures in the rock that appear to be anthropogenic, but that are commonly interpreted as natural structures.
Acknowledgements
Special thanks are addressed to Prof. Dr. Gert Goldenberg for having allowed the visit of St. Ulrich mine. Many thanks go deeply to Prof. Dr. Ünsal Yalçin (Deutsches Bergbau- Museum Bochum DBM) for having hosted the research of A. Celauro in the DBM, and Prof. Dr. Michael Prange for having supported analytical phases in the DBM laboratories. A very special and deep thank goes to Andreas Ludwig for the perfect preparation of the specimens for the optical microscope, and Georg Wange for the dedicated setting of ultrasonic measurements and the consultation on chemical issues in the fire-setting system, Dr. Stephen Merkel for having conducted very keenly the observations with optical microscopy, Dr Katrin Hitzler and Jan Sessing for the very helpful executions of the XRD analyses. Dr. Tilde De Caro is deeply thanked for the interesting preliminary considerations for setting of Raman analyses. We thank the reviewers of the paper for their helpful comments and suggestions.
Bibliography
Note: References for other basic geochemical texts, not referenced within this, can be requested from the authors.
Allison, R.J. and Bristow, G.E., 1999. The effects of fire on rock weathering: some further consider- ations of Laboratory experimental simulation. Earth Surface processes and Landforms, 24, pp.707-713.
Barker, C. and Robinson, S. J., 1984. Thermal release of water from natural quartz. American Mineralogist. 69 (11-12), pp.1078-1081.
Bates, J. B.and Quist, A. S., 1972. Polarized Raman Spectra of β-Quartz. The Journal of Chemical Physics, 56, p.1528.
Bishambhar, D. S. and Hari N., 1949. ‘Raman and infra-red spectra of b quartz’. Nature. 164, pp.583-584.
Brotons, V., Tomas, R., Ivorra, S. and Grediaga, A., in press 2018. Relationship between static and dynamic elastic modulus of calcarenite heated at different temperatures; the San Julian’s stone. Bulletin of Engineering, Geology Environment.
Burstein, M., (trans.) 1990. Agatharchides of Cnidus - On the Erythraean Sea. London.
De Caro, T., Ricucci, R., Parisi, E.I.M., Renzulli, A., Del Moro, S., Santi, P. and Faraldi F., 2013. Ar- chaeo-metallurgical studies of tuyeres and smelting slags found at Tharros (north-western Sardinia, Italy). Journal of Applied Physics A. 113(4), pp.933-943.
Craddock, P.T., 1992. A short history of fire-setting. Endeavour, 16(3), pp.145-150.
Crew, P., 1990. Fire-setting experiments at Rhiw Goch. In: P. Crew and S. Crew eds, Early Mining in the british isles, Proceedings of the mining Workshop at Plas Tan y Bwlch Snowdonia National Park Study Center, 1989, Occasional Paper 1, pp.53-54.
Deal, K. 2012. Fire effects on flacked stones, ground stone, and other stone artifacts. USDA Forest Service Gen. tech. Rep. RMRS-GTR-42, 3, pp.97-110.
Eitzenberger, A. 2012. Wave Propagation in Rock and the Influence of Discontinuities, doctoral thesis. Luleå University of Technology.
Goudie, A.S., Allison, R.J. and McLaren, S.J., 1992. The relations between Modulus of Elasticity and Temperature in the Context of the experimental simulation of rock weathering by fire. Earth Sur- face processes and Landforms, 17, pp.605-615.
Heaney, P.J. and Veblen D.R. 1991. Observations of the a-b phase transition in quartz: a review of imaging and diffraction studies and some new results. In American Mineralogist. 76, pp.1018- 1032.
Hoover H.C. and Hoover L.H. trans. 1950. Agricola G. 1556. De Re Metallica, New York
< https://www.engineeringtoolbox.com/specific-heat-fluids-d_151.html >
Kazuaki, I., 1975. Study of the Force Field and the Vibrational Normal Modes in the a-p Quartz Phase Transition. The American Mineralogist. 60, pp.907-912.
Lama, R.D., Vutakuri, V.S. and Saluja, S.S., 1978. Handbook on Mechanical Properties of Rocks 1 ,2 , 3 , 4. Trans Tech Publications
Lüttge, A. and Metz P., 1993. Mechanism and kinetics of the reaction: 1 dolomite + 2 quartz = 1 diopside + 2 CO2: a comparison of rock-sample and of powder experiments. Contrib. Mineral Petrol 115, pp.155-164.
Mahfujul, I., 2011. Polarized raman spectra of alpha quartz, thesis Institut de mineralogie et de phy- sisque des milieu condenses. Universitè Pierre et Marie Curie.
Nordlund, E., Zhang, P., Dineva, S., Saiang, C. and Mainali, G., 2014. Impact of fire on the stability of hard rock tunnels in Sweden. BeFo Report 136, Stockholm.
Picking, J. and Timberlake, S., 1988. Stone hammers and fire-setting: a preliminary experiment at Cwmystwyth mine, Dyfed. Bulletin of the Peak District Mines Historical Society, 10 (3), pp.165-167.
Py, V. and Ancel, B., 2006. Archaeological experiments in fire-setting: protocol, fuel and anthracologi- cal approach. Oxford: BAR International Series, pp.71-82.
Saiang, C., 2011. Influence of heat on physical and mechanical properties of selected rock types. Master Thesis. Luleå University of Technology, Luleå.
Sanjurjo, J., Gomez-Heras, M. and Polymeris, G., 2013. Estimating maximum temperatures at- tained during fires in building stoneworks by thermoluminescence: A case study from Uncastillo, Saragossa (Spain). Mediterranean Archaeology and Archaeometry, 13(3), pp.145-153.
Timberlake, S., 1990a. Review of the Historical Evidence for the Use of Fire-setting. In: P. Crew and S. Crew, eds. Early mining in the British Isles. Proceedings of the Mining Workshop at Plas Tan y Bwlch Snowdonia National Park Study Centre, 17-19 Nov.,1989, Occasional Paper 1, pp.49-52.
Timberlake, S., 1990b. Fire-setting and primitive mining experiments, Cwmystwyth. In: P. Crew and S.Crew eds. Early mining in the British Isles. Proceedings of the Mining Workshop at Plas Tan y Bwlch Snowdonia National Park Study Centre, 17-19 Nov., 1989, Occasional Paper 1, pp.53-54.
Willies, L. and Weisgerber G., 2000. The use of fire in prehistory and ancient mining-fire-setting. Paleorient. 26(2). pp.131-149.